Fluid aeration can cause numerous problems in a hydraulic and lubrication oil system including: unacceptable noise, poor component response due to spongy behavior of aerated fluids, cavitation damage and severe fluid degradation.
A bubble removal device can be used to mechanically remove bubbles from fluids, which not only solves these problems, but also offers advantages such as the possibility of smaller reservoir size and lower overall system cost. This article describes the principles and operation of a new device that can be installed in a fluid circuit to physically remove bubbles from the fluid during system operation.
|
|
Initial Bubble |
Initiation of Bubble Collapse |
|
|
Forming of Liquid Jet |
Impact and Metal Extrusion |
Figure 1. Cavitation Damage |
Cavitation occurs when the pressure acting on a fluid is below the saturation pressure of the dissolved gas in the fluid. At this point, if the bubbles which are flowing with the fluid as it passes through the system encounter a region of higher pressure, they will collapse as illustrated in Figure 1.
The process may be violent, depending upon the load pressure on the hydraulic pump. This can cause broad, high-frequency vibrations, noise, material damage and thermal degradation of the oil.
In addition, bubbles in the reservoir may be concentrated at the gas-liquid interface as foam or they may be dispersed or entrained within the fluid (Figure 2).
![]() |
Figure 2. Foam and Entrainment |
If bubbles are present in the fluid in the reservoir, they may be sucked into the pump, where the bubbles’ volume will increase, due to pressure decrease along the suction line, and then compress again when a region of higher pressure is encountered.
This compression is nearly adiabatic (the bubble gets hot, but does not materially increase the temperature of the surrounding fluid), resulting in tremendous localized temperatures at the gas-liquid interface. This may cause thermal cracking and sludge/varnish formation.
It can be difficult to distinguish between these two processes in field applications; therefore, both will be considered as a single cavitation process for the purpose of this discussion.
Various sources of bubble formation within the hydraulic system include:
- Suction resistance
- Pressure drop through an orifice
- Pressure drops through pipes and hoses
- Turbulence from valve opening and closing
- Shock waves due to sudden closing of valves and sudden cessation of pump operation
- Pressure drop due to sudden opening of a valve
- External force on piston rod
- Plunging of fluid at the return to tank
- Inadequate net positive suction head available (NPSHA) relative to the net positive suction head required (NPSHR) in centrifugal pumps
- Suction-side recirculation to sub-best efficiency point (BEP) operation of centrifugal pumps
- Nearly dry operation of a pump due to insufficient fluid volume.
In gear and bearing lubrication, bubbles may be created by a churning effect of the fluid as it flows through the bearing assembly. There are undesirable physical and chemical effects that result from these processes. For example, an increase in system noise, known as the water-hammer effect, typically accompanies cavitation.
In addition, cavitation may result in increased oil oxidation rates.1 When an air-ignitable mixture is present within the bubble, ignition may occur from the rise in temperature accompanying the compression process.2,3 This process requires only nanoseconds and the localized temperature may be 2012ºF (1100ºC) or higher.
This process, also known as the micro-dieseling effect may lead to oxidative degradation of the hydraulic oil, localized hot spots and pressure spikes, and may subsequently lead to cavitation erosion in a hydraulic pump or other component.4 In addition to these well-known processes, cavitation may lead to the formation of reactive chemical intermediates, which are capable of affecting secondary oxidation and reduction processes.5
These and other hydraulic system problems resulting from the presence of bubbles in a hydraulic fluid include:
- Oil temperature rise
- Deterioration of oil quality
- Degradation of lubrication (due to either viscosity loss or sludge and varnish formation)
- Reduced thermal conductivity
- Cavitation and erosion
- Noise generation
- Reduced bulk modulus (due to fluid aeration leading to a spongy fluid and sluggish system control)
- Decreased pump efficiency
- Reduced dielectric properties
Recently, Opus System, Inc. developed a device to mechanically remove bubbles from aerated hydraulic fluids called the Bubble Eliminator.
How It Works
The device, shown in Figure 3, consists of a tapered tube that is designed such that a chamber of circular cross-section becomes smaller and then connects with a cylindrical straight tube chamber. Fluid containing bubbles flows tangentially into the tapered tube from an inlet port and generates a swirling flow that circulates the fluid through the flow passage.
The swirling flow accelerates as the radius decreases, reducing the fluid pressure along the central axis as the fluid moves downstream by Bernoulli’s equation. At the end of the tapered tube, the swirl flow decelerates downstream and the pressure recovers as the fluid moves to the outlet. In a sense, this is a fluid-flow driven centrifuge action for bubble removal.
There are certain position-dependent centrifugal forces created in all parts of the swirl flow, and the bubbles tend to move toward the central axis of the device due to the difference in centrifugal force. Small bubbles are trapped, creating an air column in the vicinity of the central axis of the swirling flow near the area where the pressure is the lowest. When backpressure is applied at the downstream side of the device, the collected bubbles will be ejected through the vent port.
![]() |
Figure 4. Air Bubble Removal, |
Figure 4 illustrates the ability of the device to remove air bubbles when used with a severely aerated hydraulic fluid.
The device is manufactured in four configurations shown in Figure 5: standard, in-line, coater and the sanitary-type designs to facilitate installation and use in a variety of hydraulic circuits.
![]() |
![]() |
![]() |
![]() |
Figure 5. Configurations Include A) Standard, B) In-line, C) Coater and D) Sanitary. |
Use with Feed Pump
If the circulation circuit on the suction side of the system’s pump is placed downstream of the device, then theoretically, bubbles can be eliminated. By means of adjusting the circulation flow, the delivery flow can be controlled for the next processing stage.
If a throttle valve is incorporated on the suction side of the hydraulic system’s pump and is adjusted to decrease the pressure between the throttle valve and pump, the capacity for dissolved gas in the fluid is decreased. Thus, the fluid becomes oversaturated with the dissolved gas and bubbles come out of the fluid at the suction side of the pump. They are then fed to the device and removed from the system. When dissolved gas is decreased, the rate of gas dispersion into the fluid is increased so that fluid absorbs more bubbles, which can then be dissolved into the oil. For some chemical fluids, this method is not suitable.
Connection to Return or Processing Line
The Bubble Eliminator may also be connected to the return line of the chemical feed pump, where it may:
- Improve surface quality
- Prevent uneven spreading
- Prevent defects in quality of products
- Improve flow rate measuring accuracy
- Decrease waste material
- Increase productivity.
It has been shown that the Bubble Eliminator can effectively remove bubbles from hydraulic fluids in various hydraulic systems, which may cause cavitation damage, increased and objectionable noise, accelerated fluid degradation and other operational problems. Furthermore, in off-highway equipment design, there are ever-increasing motivations to save space and cost by using smaller reservoir designs. The use of this type of device with off-highway hydraulic systems facilitates this practice because in addition to allowing smaller reservoir size, the following advantages are achieved:
- Lightweight, smaller space, lower cost
- Slows fluid degradation, thus extending usable life of fluid
- Prevention of pump cavitation and noise
- Less fluid in a reservoir, less possibility of fire
- Less heating time in cold weather
- Decrease in fluid compressibility and increase in dynamic characteristics
- Ease of contamination control
- Simple configuration of reservoir with no baffle plate.
References
- Hobs, J., and McCloy, D. (January 1972). Cavitation Erosion in Oil Hydraulic Equipment. Metals and Materials, p. 27-35.
- Suzuki, R., Tanaka, Y., and Yokota, S. (1998). Reduction of Oil Temperature Rise by Use of a Bubble Elimination Device in Hydraulic Systems. Lubrication Engineering, Vol. 54, No. 3, p. 23-27.
- Backè,W., and Lipphardy, P. (1977). Influence of Dispersed Air on the Pressure Medium. Process Contamination of Fluid Power Systems, C97/76, p. 77-84.
- Yamaguchi, Y., and Shimiza, S. (1987). Erosion Due to Impingement of a Cavitating Jet. Trans. ASME, J. Fluids Engineering, Vol. 109, No. 4, p. 442-447.
- Suslick, K., Mdleleni, M., and Ries, J. (1997). Chemistry Induced by Hydrodynamic Cavitation. Journal of the American Chemical Society. Vol. 119, p. 9303-9304.
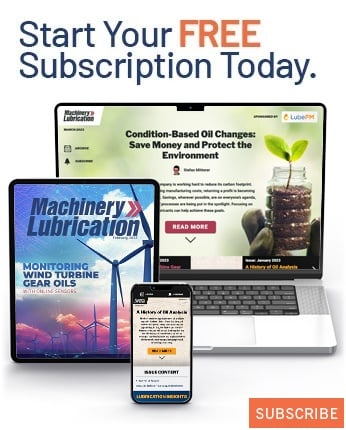