Chemical microscopy, the combination of polarized light microscopy (PLM) with micro-scale techniques of qualitative analytical chemistry, is an extremely powerful and versatile tool for solving a wide variety of contamination, particle identification and materials analysis problems.
Often neglected in used oil analysis, chemical microscopy serves as a powerful ally to other methods of analysis. In some cases, it is the only way to truly get to the bottom of a problem.
The most direct and powerful application of PLM is the identification of hundreds, if not thousands, of different types of particles and materials based only on their morphological and optical properties.
A trained microscopist can easily identify many types of fibers, pigments, food products, pollen grains, spores, hairs, glass, combustion products, pharmaceuticals, explosives, minerals and other crystalline materials by sight or with the aid of a few simple optical tests that can be conducted in a matter of seconds with the polarizing microscope.
By using the unparalleled pattern recognition capabilities of the human eyes and brain, PLM offers a degree of speed and specificity unmatched by any other analytical technique. The properties of individual particles, as small as a few micrometers, that can be determined with the PLM include: size, shape, color, density, surface texture, refractive indices, pleochroism (colors exhibited by a mineral based upon selective absorption and trasmittance of light), transparency/opacity, crystal habit, crystal system, interfacial angles, elasticity, solubility and more.
A valuable advantage of PLM is that it allows different types of particles with essentially the same chemical makeup to be easily distinguished and identified. Cellulose, for example, is perhaps the most common organic material on earth, and it occurs in many different forms, including wood and other bulk plant tissues, synthetic rayon and a variety of natural textile fibers, including cotton, paper, linen, hemp, jute and ramie.
The microscopist can easily and quickly distinguish all of these without the aid of chemical analysis. Figure 1 depicts cotton fibers, identifiable by their distinctive twisted ribbon shape. The linen fibers in Figure 2 are distinguished by characteristic cross-hatching marks. The rayon fibers in Figure 3, produced from regenerated cellulose, have an irregular cross-section, causing them to have a striated appearance when viewed with a microscope.
Conventional spectroscopic techniques such as Fourier transform infrared spectroscopy (FTIR) will show little, if any, difference between these forms. Similarly, elemental analysis techniques such as energy dispersive X-ray spectroscopy (EDS), X-ray fluorescence (XRF) and atomic emission/absorption (AAS/AES) will provide no distinguishing information about these different samples.
![]() |
Figure 1. Cotton Fibers
|
Silica is another common material that occurs in multiple forms that are easily distinguishable with the light microscope. A client recently submitted to McCrone Associates a sample of a drug product contaminated with thin, brittle black flakes. Micro FTIR and EDS analysis of the flakes showed only the presence of silica in a charred organic matrix.
Unfortunately, these techniques did not indicate which of the numerous forms of silica (such as quartz, cristobalite, opal, diatomaceous earth, perlite, pumice, silica gel or fused silica glass) was present.
When the black flakes were dispersed and examined with the polarized light microscope, it was immediately seen that the silica particles were plant phytoliths (Figure 4).
Plant phytoliths are small siliceous bodies that grow within the tissues of certain plants, including grasses, and have distinctive morphologies that correspond to the shapes of the cells in which they formed. Acting on this lead, further inquiries showed that the sample had been contaminated by ash from a recent brush fire near the client’s facility.
When microchemical and microcrystal tests are added to the repertoire of the chemical microscopist, his or her problem-solving ability expands even further. Microchemical tests rely on a color reaction when the sample is subjected to a chemical to identify a specific material or class of compounds.
Are the small red-brown specks contaminating a sample rust or degraded organic material? Treating a few of the particles with a microdrop of potassium ferrocyanide solution in hydrochloric acid will immediately identify any iron-rich particles by the deep blue precipitate of ferric ferrocyanide that is produced.
Are the colorless flakes in a dust sample bits of polymeric material or epithelial (dead skin) flakes? The stain amido black will quickly color any proteinaceous skin flakes, while leaving polymeric material unaffected.
![]() |
Figure 2. Linen Fibers
|
![]() |
Figure 3. Rayon Fibers
|
![]() |
Figure 4. Plant Photoliths
|
![]() |
Figure 5. Lead Iodide Crystals
|
![]() |
Figure 6. Borken Ends of Fiber from Auto Transmission Filter
|
Microcrystal tests, on the other hand, rely not on color changes alone, but involve the formation of minute, characteristic crystals. Microcrystal test methods typically involve dissolving a tiny crystal of the material to be tested in a microdrop of water on a glass slide, and a crystal of the test reagent in another drop.
The two drops are drawn together, allowing the solutions to mix. A positive reaction is indicated by the production of crystals of a specific color and morphology, which can be easily identified with the microscope. These tests can provide rapid and specific identification of individual particles no larger than the period at the end of this sentence.
A majority of the elements in the periodic table can be identified in nanogram quantities by these tests, which require only a few minutes to conduct. For example, a microscopic fragment of metal is suspected of being lead. Using a microtool, a portion is excised and dissolved in a small drop of dilute nitric acid.
A tiny crystal of potassium iodide is dissolved in a second droplet of water and the two droplets are drawn together. The formation of bright yellow, hexagonal plates of PbI2 (Figure 5) identifies lead specifically. A highly versatile reagent for microcrystal tests is 1,2-dihydroxycyclobutenedione, commonly called squaric acid because of the molecule’s square shape.
More than 15 different elements or ions can be specifically identified using squaric acid as a test reagent. Squaric acid can even be used to distinguish ferric (Fe+3) and ferrous (Fe+2) ions in solution, as it will give a different reaction with each.
Utilizing Filter Fiber Failure
In a study described by McCrone1, lubricating oils were found to thicken unacceptably at winter operating temperatures. By cooling samples of the oil with a cold stage on a polarized light microscope, it was seen that waxes in the oil were crystallizing into networks of long ribbons at low temperatures, reducing the viscosity.
By observing the optical properties of the wax crystals, it was determined that the ribbons grew most rapidly in the directions perpendicular to the lengths of individual paraffin chains.
With this information, it was possible to choose additives (decyl and dodecyl methacrylates) which would be adsorbed onto the more rapidly growing wax crystal surfaces, slowing growth in those directions and causing the crystals to grow thicker and wider, rather than forming the elongated chains which reduced viscosity.
In a recent project at the McCrone laboratory, a client submitted an automotive transmission fluid filter composed of polyester fibers held together with a sprayed-on resin binder. After being immersed in transmission fluid and heated to 150°C (302°F) for 200 hours, the filter had almost no tensile strength.
PLM examination of fibers from the failed filter and a normal filter showed immediately that the failure was not due to differences in fiber length, fiber diameter, polymer orientation or amount of binder between the good and bad filters, as the client had originally suspected.
Rather, it was seen that the failure was occurring in individual fibers, which were breaking with sharp, elongated tips and “stake and socket” ends (Figure 6). This type of failure is consistent with the breaking of polymer chains initially at the fiber surface followed by propagation of mechanical damage across the fibers.
This indicated that the conditions of the test might have exceeded the usual temperature parameters, causing damage that would normally not have occurred at 150°C (302°F).
In those cases where further analytical methods are required, PLM can provide valuable preliminary information on the nature of the sample, allowing the more time-consuming and expensive instrumental methods to be employed with greater efficiency.
Conclusion
Because of this, polarized light microscopy should be part of the repertoire of any laboratory involved in solving industrial problems of contamination or microanalysis. Its speed and versatility in sample evaluation and identification make it an invaluable tool that in combination with other analytical techniques can lead to solutions of problems that would otherwise be intractable.
Reference
1. McCrone, W. C. (1957). “Fusion Methods in Chemical Microscopy”. Interscience Publishers, New York, NY.
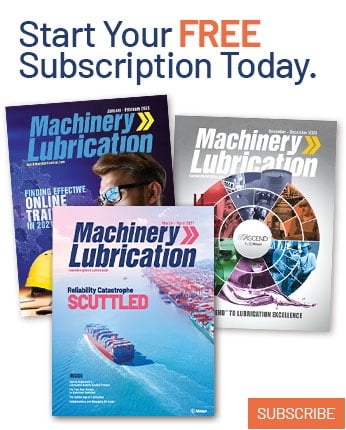