For those just getting started in the oil analysis game, the learning curve can initially seem steep and daunting. Technical jargon such as elemental analysis, Fourier Transform Infrared Spectroscopy (FTIR), absolute and kinematic viscosity, ISO particle count, wear debris analysis and Karl Fischer moisture can easily intimidate those who haven’t taken a chemistry class in many years.
So, for our oil analysis freshmen, POA will be running a series of articles throughout 2002 on all of these fundamental oil analysis tools to explain how they work, what they are designed to tell you and what all those numbers that come back from the lab actually mean. The first article in the Oil Analysis 101 series will focus on Elemental Analysis.
Elemental analysis is probably the most fundamental test in the oil analysis toolbox. Its history goes back to the 1940s and 1950s when it was used in the railroad industry to determine the presence of wear metals in diesel engine oils. However, elemental analysis, sometimes referred to as elemental spectroscopy, atomic emission spectroscopy (AES) or simply wear metal analysis is about more than just measuring the concentrations of wear metals such as iron, lead and copper.
In its present form, elemental analysis is used to determine the concentrations of 15 to 25 different elements ranging from wear metals and contaminants to oil additives. Table 1 gives an overview of some common elements that are measured and their usual source.
Elemental analysis works on the principle of atomic emission spectroscopy (AES). In AES, individual atoms within the sample, for example iron atoms from wear debris, zinc atoms from a ZDDP additive molecule, or silicon from silica (dirt) contamination, are excited using a high-energy source.
The atoms absorb energy from the excitation source and are transformed to a high-energy electronic state. Due to the laws of quantum physics, atoms do not like being in these excited states and rapidly lose the energy they have gained, mainly by emitting light energy.
The energy of light emitted, which is inversely proportional to the wavelength, is dependent on the electronic structure of the atom, and is thus different for each type of atom. So, by measuring the amount of light emitted at the characteristic emission wavelength for atoms such as iron, copper, zinc and sodium, the concentration of each atom can be determined.
Influence of Particle Size
The major limitation with AES is that because the method requires excitation of individual atoms, the sample must be fully vaporized to allow all atoms present to be measured. While this is not a problem for small particles and dissolved metals, the probability that a particle can be vaporized and analyzed using AES drops very rapidly above 5 microns. In fact an AES spectrometer is all but blind to particles in excess of 10 microns.
Unfortunately, depending on the wear mechanism and the severity of the problem, active machine wear may generate particles that are greater than 10 microns in size, and will thus be invisible to the AES instrument. For this reason, it is important in any oil analysis program to not rely solely on AES data to determine active wear, but to include tests such as particle counting, ferrous density analysis and patch microscopy to measure larger particles.
Units of Measure
So, what exactly is a ppm? On an oil analysis report, the numbers typically seen, depending on component type, oil type, application, etc., may range from a few parts per million (ppm) or several hundred ppm for wear metals and contaminants, to several thousand ppm for certain additive elements.
As the name implies, a ppm is simply the number of parts of the element in question, per million parts of the sample. Put another way, a reading of 1 ppm of a particular element is equivalent to 1 µg of the element per g of sample, which is the same as 1 mg per Kg, or approximately one ounce per 6500 gallons of oil!
How does the lab go from measuring light intensity to reporting a concentration in ppm? I would use the original form. To do this, each AES instrument is calibrated using standard calibration solutions. These solutions contain known concentrations of certain elements of interest. Metal sulfonates are commonly used for this purpose.
By analyzing the calibration standards, the amount of light at a specific wavelength can be determined for the elements in question, which can then be related to the known concentration in the standard solution.
In this way, a calibration curve can be generated which allows the unknown concentration of the same element in a test sample to be determined by measuring the amount of light emitted at a specific wavelength, and using the calibration curve to convert this light intensity into a concentration or ppm.
Trending the Data
When analyzing elemental analysis data, it is important to look not at the absolute value of each element, but rather the trend line, that is, the change in elemental concentrations over consecutive samples.
This is important because wear rates will be different for different machines, depending on component types, manufacturer and model, oil type, age, usage, etc. This type of rate-of-change analysis can be invaluable in finding early signs of wear and contamination related problems.1
In analyzing AES data, it is important to know both the machine metallurgy, and the chemical composition of common contaminants that may be present so the data can be related back to active wear of a specific component, or to ingress of specific contaminants.
It is also important to know the expected concentrations of the different metal-containing additives in the oil. To do this, new oils should be baselined on an annual basis, or whenever a change in oil type or formulation is suspected. By comparing the elemental fingerprint of the new oil baseline to the used oil sample, problems such as additive depletion or the addition of wrong oil can be quickly and easily diagnosed.
Care should be exercised however, when looking at elemental additive elements because additive depletion does not necessarily cause a drop in additive element concentration, as measured by AES.2
Due to its ability to determine unusual wear, contaminants and additive elements, AES is an invaluable tool in any oil analysis program. Used correctly, it can determine everything from corrosive wear, to a coolant leak, to sea water ingression to additive stripping and should be considered the cornerstone of a well-engineered oil analysis program.
Anatomy of an Atomic Emission Spectrometer
Nearly all oil analysis labs use one of two types of atomic emission spectrometer, either an inductively coupled plasma (ICP) instrument, or a rotating disc electrode (RDE) instrument. The basic difference between the two lies mainly in the way in which the sample is vaporized and the atoms excited by the high-energy source.
In an ICP instrument, the oil is injected into a high-temperature argon plasma, where the atoms are vaporized, excited and subsequently emit light. In an RDE spectrometer, also sometimes referred to as an “Arc-Spark” instrument, the oil is vaporized and excited using a high voltage discharge between an electrode and a rotating carbon disc.
The rest of the instrument, whether it be an ICP or RDE spectrometer, is basically the same. The light emitted by the excited atoms is collected and focused onto the slits of the spectrometer. The spectrometer contains a diffraction grating, which is similar to a prism in that it splits light of different wavelength or colors into discrete wavelength, based on their angle of diffraction.
The light intensity at each angle, typically referred to as a channel, is measured using a light-sensitive photodiode and the resultant voltage signal converted to a concentration in ppm based on a simple calibration procedure.
In practice, provided the two instruments are calibrated properly, there is very little difference between the accuracy of data from both types of instrument. However, there is one very important difference between ICP and RDE instruments. Both ICP and RDE instruments suffer from size limitation effects.
This effect limits the size of particle that can be measured using conventional AES. For ICP, only particles smaller than approximately 3 microns can be measured. For RDE instruments, the limit is slightly higher, around 8 to 10 microns. The implication is that if an oil sample is analyzed first by ICP, then by RDE, the concentrations of certain elements, particularly wear metals and contaminants, which may be present as 3- to 10-micron particles will potentially be different.
While this is of little concern when trending data from different samples analyzed by the same instruments, data from samples analyzed by RDE and ICP instruments typically will not correlate.
References
1. Sowers, J. (2001). “Use Statistical Analysis to Create Wear Debris Alarm Limits.” Practicing Oil Analysis. November-December, p. 38-41.
2. Gustavsen, A.J. (2001). “Assessing Additive Health Using Elemental
Spectroscopy and the Stoke’s Procedure.” Practicing Oil Analysis. May-June, p. 14-16.
Further Reading
1. “Source Book For Used Oil Elements,” by James C. Fitch (available in the Bookstore at www.practicingoilanalysis.com).
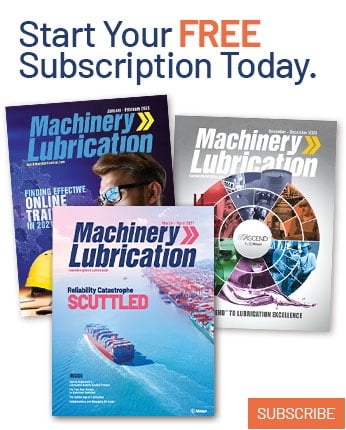