The use of biogas engines and their maintenance has become a hot topic over the last few years. Biogas is a renewable fuel, so it qualifies for renewable energy subsidies in some parts of the world.
Biogas typically refers to a gas produced by the biological breakdown of organic matter in the absence of oxygen. It is this type of gas that can be used as biofuel.
One type of biogas is produced by anaerobic digestion or fermentation of biodegradable materials such as biomass, manure, sewage, municipal waste, green waste and energy crops. Via this formation, biogas is produced that consists primarily of methane and carbon dioxide.
Another possible source of biogas is wood gas, which is created by gasification of wood or other biomass. This type of biogas is comprised primarily of nitrogen, hydrogen and carbon monoxide with trace amounts of methane.
As a renewable energy source, biogas can be utilized for electricity production on sewage works in a combined heat and power (CHP) gas engine. If compressed, it can replace compressed natural gas for use in vehicles, where it can fuel an internal combustion engine.
The methane, hydrogen and carbon monoxide gases can be combusted or oxidized with oxygen. Air contains 21 percent oxygen. This energy release allows biogas to be utilized as a fuel. Biogas can be used as a low-cost fuel in any country for any heating purpose, such as cooking.
It can also be employed in modern waste-management facilities where it can be used to run any type of heat engine and generate either mechanical or electrical power.
Landfill gas typically has methane concentrations around 50 percent. Based on advanced waste-treatment technologies, biogas can be produced with 55 to 75 percent methane. The chemical composition of biogas varies depending upon the origin of the anaerobic digestion process.
Methane within biogas can be concentrated via a biogas upgrader to the same standards as fossil natural gas, becoming bio-methane. Carbon dioxide, water, hydrogen sulfide and particulates (such as siloxanes) must be removed if present. These siloxanes are formed from the anaerobic decomposition of materials commonly found in soaps and detergents.
During combustion of biogas-containing siloxanes, silicon is released and can combine with free oxygen or various other elements in the combustion gas. Deposits are formed containing mostly silica or silicates as well as calcium, sulfur, zinc and phosphorus.
These white mineral deposits accumulate to a surface thickness of several millimeters and must be removed by chemical or mechanical means.
In comparison to natural gas engines, the operating conditions of biogas engines are different because of higher combustion temperatures, 24/7 operation to avoid methane release and contamination from carbon dioxide, water, hydrogen sulfide (acidic) and particulates (siloxanes).
In general, gas engines are used to power cogeneration electrical power plants and are preferred over diesel engines because of their lower exhaust emissions and lower fuel cost.
Formulation Changes for Gas Engine Oils
Gas engine oils used in biogas or natural gas engines have special formulations that differ from diesel and gasoline engine oil formulations. This is because natural gas engines have:
- A clean burning process with no soot contamination. Therefore, the oils require less detergency/dispersancy (lower ash levels).
- A gaseous fuel source with no risk of fuel dilution. The prevention of a viscosity increase in the oil is more critical.
- A hotter burning process, which is typically from 165 to 235 degrees C. Thus, oxidation/nitration is of greater concern. (The heat may be caused by hot spots within the oil’s circulation located near the piston rings and the combustion chambers.)
For gas engines, the goal is to obtain a lubricant with higher thermal and oxidative stability or to create a lubricant with a minimal oxidative degradation. Standard results for lubricants with poor oxidation protection/control are:
- Sludge formation/filter blockage
- Oil thickening /increased viscosity
- Formation of deposits/oxidation products such as lacquers, varnishes, etc.
- Increased acidity
These operating problems can be prevented by the combination of a good quality base oil with a supplemental antioxidant package. A typical gas engine oil formulation may contain multiple antioxidant additives to help protect the base stock from excessive heat or catalytic degradation.
Antioxidants added alone or in synergistic combinations extend the operating life of the oil by improving its oxidation stability. During their use in lubrication systems, oxidation inhibitors will deplete to a certain critical level, at which point the fluid will start to degrade/polymerize at an accelerated rate.
When this happens, important changes in the physical properties of the base stock occur. The oil or lubricant is no longer able to protect the equipment, and its useful life is over.
There is a growing interest in methods predicting an oil change by measuring antioxidant concentration during the lubricant’s lifetime. Monitoring the onset and the propagation of oxidation will lower operating costs and allow users to detect abnormally oxidized and degraded lubricants.
The basic mechanism of oxidative degradation and the role that different antioxidants play begin with the formation of reactive compounds, better known as free radicals, hydro peroxides and peroxides. These reactive compounds propagate into oxygenated hydrocarbons, such as alcohol, acids and sludge.
The antioxidants act in two ways: by removing radicals (primary antioxidancy) or by decomposing hydro peroxides to form non-reactive products, which do not participate in further oxidation of the lubricant.
Currently, the most commonly used primary antioxidants are the phenolic, phenates, salicylates and amine types; the secondary antioxidants are usually sulfur or metal-containing additives.
Once the antioxidant additives are depleted, the base oil is more vulnerable to oxidation. This may explain why the oil’s viscosity tends to increase as more operating hours accumulate on the gas engine.
Figure 1 shows a RULER voltammogram for different gas engine oil formulations. As you can see, the selection of different types of antioxidants is applied for the gas engine oil formulations, where the following types of antioxidants are used: Zincdithiophosphates (ZnDTP), aromatic amines, phenates, phenols and salicylates.
Figure 1. Multi RULER graphs representing different gas engine oil formulations.
Relationship between Antioxidants and Viscosity
Actual oil analysis specifications by acid number and base number in combination with elemental analysis and contamination are no longer sufficient to define the correct oil change intervals or detect changing operating conditions.
One of the innovative oil analysis techniques applied and selected for these oil diagnostic programs is individual antioxidant monitoring by linear sweep voltammetric techniques (RULER technology).
By monitoring individual antioxidants, in comparison to acid number, operators will be able to establish an accurate condition of the oil as part of the oil’s lifecycle evaluation.
Figure 2. Correlation between phenolic depletion (FTIR) and viscosity increase.
Advantages of Different Methods for Oil Lifecycle Evaluation
Over the years, oil change frequencies or drain intervals have been recommended by each engine manufacturer. These can vary from 500 to 1,500 operating hours. The use of contaminated biogases along with high-load factors on the engines can significantly reduce these drain intervals.
However, they can also be extended with good maintenance and operational practices, as well as oil monitoring through an adapted in-service oil analysis program. This is particularly important if the quality of the gas is unsteady.
The results of the following experimental program will show how the exact combination of the selected oil analysis parameters can lead to a high value and sophisticated oil diagnostics program.
Case Study #1 – DEUTZ Biogas Engine
This study occurred at a site where vegetable green waste was generating methane through fermentation and driving the eight DEUTZ biogas engines using an oil formulation typically formulated for biogas engine operations. Figure 3 shows the analysis for an in-service oil sample after 300 hours of operation.
Figure 3. RULER graph for biogas engine oil from the DEUTZ engine after 300 operating hours.
Based on different cycles for the same engine, the oil was closely monitored every 100 hours for the following oil parameters: viscosity (100 degrees C), AN, BN, oxidation, percent water volume, flash point, detergency, glycol, elements, chlorine, antioxidants and oil top-up volume.
In addition, boroscopic control information was made available, which showed no increased deposits in the critical areas of the engine.
From this data, four critical oil parameters were selected: antioxidants, AN, BN and oxidation (by FTIR). The correlation was indicative of the antioxidants’ value:
- Antioxidant #1 depleted within 50 percent of the lifecycle, followed by a depletion of antioxidant #2. It was noted how antioxidant #2 remained in concentrations higher than 50 percent.
- The acid number’s increase was less predictable but showed the highest number with the lowest remaining antioxidant concentrations.
- AN and BN did not cross each other.
- Oxidation by FTIR increased gradually over the lifetime cycles.
Figure 4. Correlation between antioxidants, AN, BN and oxidation by FTIR for DEUTZ biogas engines.
Case Study #2 – Jenbacher Biogas Engine
Figure 5 shows the RULER analysis for in-service oil samples between 500 and 2,246 hours of operation, indicating a zinc-free additivated oil (consisting of two different types of ashless/metal-free antioxidants).
Figure 5. RULER Multigraph for biogas engine oils in service on Jenbacher engines.
The RULER voltammogram depicts the depletion of two antioxidants, in this case an aminic and a phenolic formulation over 2,246 hours.
Based on different cycles for the same engine, the oil was closely monitored every 300 hours for the following oil parameters: viscosity (40/100 degrees C), AN, BN, i-pH-value, oxidation, nitration, percent water volume, detergency, glycol, elements, antioxidants and oil top-up volume.
From the data, representing 2.246 operating hours, five oil parameters were selected: antioxidants (for two individual antioxidants), AN, BN, viscosity at 40 degrees C and at 100 degrees C. The correlation was indicative of the antioxidants’ value. In the graph below, an overview of these parameters can be found:
- Antioxidant #1 and #2 depleted down to a value below 20 percent of the fresh oil value.
- The acid number showed the highest number with the lowest remaining antioxidant concentrations.
- AN and BN did not cross but were very close to each other at the end.
- A viscosity increase was found. The viscosity at 100 degrees C was very close to 18 mm²/s, which is the limit for several engine manufacturers.
Figure 6. Correlation between antioxidants, AN, BN and viscosity for Jenbacher biogas engines.
Case Study #3 – Jenbacher 612 Natural Gas Engine
Figure 7 shows the RULER analysis for in-service samples between 1,600 and 2,000 hours of operation, indicating an additivated oil (consisting of two different types of antioxidants).
Figure 7. RULER multigraph for natural gas engine oils for an in-service Jenbacher engine.
The RULER voltammogram above reveals a clear depletion of the two antioxidants, in this case an aminic and a salicylate formulation. The yellow line, which marks the highest amount of operating hours of the oil, shows a slightly higher amount of antioxidants compared with the previous sample because there was a refilling of a higher amount of oil between the two samples.
Based on different cycles for the same engine, the oil was closely monitored every 200 hours for the following oil parameters: viscosity (40/100 degrees C), AN, BN, i-pH-value, oxidation, nitration, percent water volume, detergency, glycol, elements, antioxidants and oil top-up volume.
From this data, representing 1.964 operating hours, three oil parameters were selected: antioxidants (for two individual antioxidants), viscosity at 100 degrees C and i-pH-value. The correlation was indicative of the antioxidants’ value. In the graph below, an overview of these parameters can be found:
- The degradation of the two antioxidants was not equal. The main antioxidant in this formulation (antioxidant #2) showed a value of about 50 percent remaining antioxidant level, while antioxidant #1 had only a value of about 20 percent remaining antioxidant level.
- Viscosity at 100 degrees C showed a slight increase.
- AN and BN did not cross each other.
- The i-pH-value sank under the limit (4.00) of biogas engines.
Figure 8. Correlation between antioxidants, i-pH value and viscosity for Jenbacher natural gas engines.
Case Study #4 – Waukesha Natural Gas Engine
Figure 9 shows the RULER analysis for in-service oil samples between 1,600 and 2,000 hours of operation, indicating an additivated oil (consisting of three different types of antioxidants).
Figure 9. RULER multigraph for natural gas engine oil on in-service Waukesha engines.
The RULER voltammogram above depicts good depletion of the two main antioxidants – an aminic and a salicylate formulation. There was also a third antioxidant, which was a phenolic formulation.
Based on different cycles for the same engine, the oil was closely monitored for nearly 4,000 hours for the following oil parameters: viscosity (40 degrees C/100 degrees C), AN, BN, i-pH value, oxidation, nitration, percent water volume, detergency, glycol, elements, antioxidants and oil top-up volume.
From this data, three oil parameters were selected: antioxidants (for two individual antioxidants), viscosity at 100 degrees C and i-pH value. The correlation was indicative of the antioxidants’ value. In the graph below, an overview of these parameters can be found:
- The degradation of the two antioxidants was not equal. The main antioxidant in this formulation (antioxidant #2) showed a value of about 40 percent remaining antioxidant level, while antioxidant #1 had a value of about 55 percent remaining antioxidant level.
- Antioxidant #3 had a value of about 15 percent remaining antioxidant level.
- Viscosity at 100 degrees C showed a slight increase.
- AN and BN did not cross each other.
- The i-pH value was below the limit of 4 for a longer period of time. This correlated to the phenolic antioxidant, which was below 25 percent, since the time the i-pH value crossed the line of 4.
Figure 10. Correlation between antioxidants, i-pH value and viscosity for Waukesha natural gas engines.
Alternative Methods to Determine Oxidation
Traditionally, the oxidation number in used gas engine oils is measured by FTIR at the wave length of 1,710. Nearly every gas engine manufacturer has a limit for oxidation number based on this accepted method. Most manufacturers have set their limit value for this number at 20 A/cm.
In some modern gas engine oils, you can find components of base oil and additives that have one or more fresh oil peaks in this range (about wave length 1,710). This will complicate the interpretation of the oxidation number.
Figure 11. Example of how the oxidation number is determined in a modern gas engine oil.
Figure 12. Another example of how the oxidation number is determined in a modern gas engine oil (trend during 750 hours).
Two main effects of the DIN-oxidation (DIN 51453) method are that an oxidation value of more than 10 can initially be detected in the fresh oil and that the oxidation products are running against the additive depletion. Figure 12 shows that influence of the additive depletion in this area is so strong that it seems as if the oxidation in the spectra is lowering in the trend. In this example, an increasing oxidation number up to 22 A/cm was found followed by a decrease to 17 A/cm. Most of the gas engine manufacturers specify a maximum limit of 20 A/cm for an oil change. In this case, it was obvious that this limit made no sense for such engine oil formulations.
To obtain comprehensive information about oil oxidation with such a modern formulation, it is not possible to work with this traditional DIN method. Even in DIN 51453, it states that the interpretation of the results analyzed with this standard is impossible in oils with ester-containing additives or in ester-based fluids.
One possibility to get an idea of the running oxidation process in the oil in use is to watch the depletion of the phenolic inhibitor, which is not only detectable with the RULER but also with FTIR.
Figure 13. FTIR spectra of one modern gas engine oil.
Figure 14. Detail of the phenolic inhibitor during 750 hours in use.
Although it is possible to observe the phenolic antioxidant in the FTIR spectra, only one antioxidant can be monitored well. Therefore, another possibility was sought to check the oxidation of the used oil in the engine.
An ASTM method for the determination of oxidation (ASTM D 7214-06), which includes a special chapter for ester-containing fluids, may be a good alternative to DIN 51453. At the moment, many gas engine oils are being compared using this ASTM method with the corresponding results of viscosity increase, DIN oxidation, AN, BN and phenolic antioxidant. It is recommended to replace the DIN oxidation method for used gas engine oils with a more comprehensive method for the detection of the real oxidation of modern oil formulations.
In conclusion, it was determined that antioxidant analysis can be useful both for small and large oil reservoirs, as well as peak/base-load operations. The importance of individual antioxidants in combination with other critical parameters may provide a better understanding of the processes occurring during the oil’s lifetime. In addition, oil analysis can be achieved as part of an improvement of oil lifecycle estimation as well as equipment reliability and availability. However, for the monitoring of oxidation, especially for smaller gas engines, it is useful to seek a better method than the traditional DIN oxidation method.
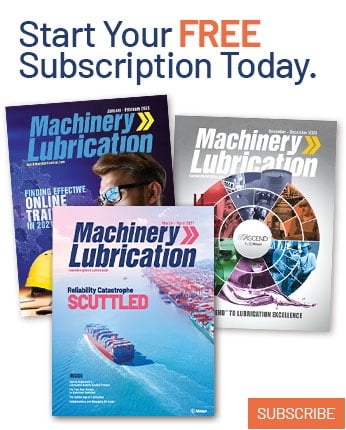