Historically, we have employed oil analysis to identify lubricant oxidation by trending the acid number (AN), Fourier transform infrared (FTIR) oxidation and viscosity.
Once evidence of oxidation is discovered, an oil drain is scheduled and, where appropriate, root cause analysis performed if the degradation cycle is too short or if we intend to extend the service interval by upgrading the oil. It seems like a reasonable approach oil analysis drives the decision.
However, in the interest of avoiding the negative consequences associated with oxidized oil, including corrosion and varnishing, and to facilitate better maintenance planning and scheduling, wouldn’t it be preferable to employ oil analysis to evaluate a lubricant’s ability to resist oxidation or measure its remaining useful life (RUL)? The concept of measuring a lubricant’s remaining useful life is not new, but its application has historically been limited to large-volume systems.
However, newer technology, called Linear Sweep Voltammetry (LSV), significantly reduces the cost of performing RUL measurements making this a viable test for even the smallest volume system. If adopted, LSV could forever change the manner in which lubricant oxidation is managed and routine used oil analysis is performed.
While the LSV method has been available in commercial form for a number of years, this technology has recently been standardized by the American Society for Testing and Material (ASTM) in standard D6810-92, and is to be further standardized in ASTM D6971, lending credibility to its purported validity and usefulness. The technology can support routine RUL measurement for a wide variety of plant equipment within external laboratory-based or on-site instrument-based oil analysis programs.
Measuring Remaining Useful Life
As the oil degrades, a sequence of events occurs, each of which can be measured with oil analysis. At first, the antioxidant additive package depletes. Then, the base oil oxidizes (Figure 1). Oil oxidation is a series of chemical reactions both initiated and propagated by reactive chemicals formed within the oil called free-radicals. The antioxidant additive is sacrificial - it is there to protect the base oil from oxidation.
The most common antioxidant additive, a phenolic inhibitor, works to neutralize the free-radicals that cause oxidation while aromatic amines, another commonly used class of antioxidant additives, work to trap free-radicals. Some antiwear additives, such as zinc dialkyldithiophosphate (ZDDP), pull double duty as antioxidants. They decompose peroxides formed as a by-product of oxidation and other chemical reactions. Over time, the antioxidant additives deplete to the point where they can no longer effectively protect the base oil.
Figure 1. Measuring Remaining Useful Life
Monitoring the oil’s ability to resist oxidation with RUL trending occurs in the proactive monitoring domain, as described in Figure 1. Working in the proactive domain, the lube tech can perform a partial drain and fill or top-treat the oil to replenish the antioxidant concentration to avoid base oil degradation. (Author’s note: Additive replenishment should be undertaken with extreme care and with the advice of properly skilled lubricant experts.)
Likewise, for planning and scheduling purposes, RUL monitoring provides management with a significant forewarning of impending oil failure (assuming no intervention to affect the chemistry), which allows the event to be handled in such a way that cost and impact on the organization are minimized.
Once the additives have depleted and base oil oxidation starts, the opportunity to be proactive expires, and one moves into the responsive domain (Figure 1) where the lubricant must be changed or significantly treated to avoid unwanted consequences such as sludging, varnishing, sticking valves, corrosion, etc.
Conventionally, used oil analysis has focused on measuring the by-products of base oil oxidation, such as acids formed as a consequence of oxidation, not on the oil’s ability to resist oxidation. For example, the AN test employs a potassium hydroxide (KOH) reagent to neutralize acid in the oil. The volume of alkaline reagent required to reach the point of neutralization is a function of the concentration of acid in the oil.
The premise is that when the oil oxidizes, organic acids are produced and collect in the oil, causing the AN to rise. Another technique employed to detect base oil oxidation is FTIR analysis. FTIR analysis effectively measures the concentration of various organic or metallo-organic material present in the oil. When oil is oxidized, the hydrocarbon oil molecules can become recompounded into soluble and insoluble oxidation by-products. FTIR measures the accumulation of these by-products.
Viscosity change is usually a lagging indicator of oxidation. As the oil degrades, the average molecular weight increases, producing an increase in viscosity. Despite the validity of all of these measurements, the fact remains that they all reveal damage to the base oil after it has occurred, which is not ideal because associated maintenance must, by definition, be responsive. A preferable scenario is to measure and evaluate the oil’s ability to resist oxidation, its RUL, which facilitates proactive lubricant maintenance.
Measuring RUL is not a new concept. However, due to the complexity and cost of performing the test, its application has been limited to highly critical, large-volume systems such as turbine generators. For years, numerous methods have been available for measuring RUL. The most common of these tests is the rotating pressure vessel oxidation test (RPVOT) - ASTM D2272, formerly called the rotating bomb oxidation test (RBOT).
In essence, the test involves placing a sample of oil into a pressure vessel along with a ration of water and a copper coil. The vessel is pressurized to 90 psi with pure oxygen and placed in a heating bath set at 150°C on a device that rotates at 100 rpm. As the temperature of the pressure vessel and its content increases, the pressure increases. The point at which it stabilizes is defined as T0, which represents the start of the test.
Over time, the oil’s ability to resist oxidation degrades as a result of stress-induced additive depletion, to the point where the base oil starts to react with the oxygen as the oil molecules begin to oxidize. Because the oxygen is being incorporated into the oil’s chemistry, the pressure within the sealed pressure vessel begins to drop. The point at which the pressure drops by 25 psi from the pressure at T0 defines the termination of the test, denoted as T1. The time, in minutes, required to go from T0 to T1, is reported as the oil’s RPVOT value (Figure 2).
As the additive package degrades, the base oil is afforded less protection. As a result, the number of minutes required to reach T1 declines as an oil begins to age in-service, indicating a loss of RUL. Of more interest is the actual RUL, which is a function of the in-service sample’s RPVOT value relative to the baseline value for the new oil, before it went into service.
The RPVOT test is reliable, but it is time consuming, costly and requires a great deal of direct supervision. Its results can also be misleading under certain circumstances. As a result, the RPVOT test is not suitable for a wide cross-section of lubricated machines in the plant.
Figure 2. Rotating Pressure Vessel Oxidation Test (RPVOT)
Several years ago, work was undertaken at the University of Dayton by Robert Kauffman to develop an easier way to measure the oil’s RUL. This research resulted in what is now referred to and standardized in ASTM D6810-02 as LSV. To conduct the test, the analyst simply adds a measured sample of oil to a vial containing an electrolyte solution. The analyst then voltammetrically analyzes it to determine the concentration of antioxidant by weight or by millimoles (mmol).
The voltammeter exposes the sample and electrolytic solution to variable sweep voltage, which causes the additives to electrochemically oxidize. The current passing between the electrodes is a function of the additive concentration. A drop in the amplitude of current as compared to the baseline amplitude at the voltage of interest is indicative of depletion of the antioxidant additive (Figure 3).
Figure 3. Depletion of the Antioxidant Additive
While ASTM D6810 specifically targets the measurement of phenolic inhibitors in turbine oil, ASTM has approved a second standard, ASTM D6971, to measure the concentration of phenolic and aromatic amine antioxidants in nonzinc turbine oils.
The instrument supplier claims that the method is perfectly capable of measuring oxidation resistance provided by ZDDP and similar antioxidant/antiwear additives, but has elected not to pursue standardization of an associated test procedure due to waning use of zinc-based additives.
Perhaps as interest grows in applying the technology to a wider range of industrial lubricants, there will be renewed interest in creating additional standard test methods. Details about the methods and available instruments to carry them out are available elsewhere in the literature. The bottom line is that the test can be performed in a matter of seconds and the cost per sample is in line with the cost for other routine used oil analysis tests.
There is compelling evidence that LSV can effectively and efficiently estimate an oil’s RUL. Many comparisons to RPVOT and other conventional measures of RUL have been conducted, and the instrument seems to perform reliably.
The ASTM committee overseeing such tests was sufficiently convinced of the technique’s validity to issue a standard related to its use - and they are a tough crowd of highly knowledgeable scientific experts. Of more urgent interest to the plant lubrication or reliability engineer is the question about how to put LSV to work.
Why Include RUL Monitoring In Your Oil Analysis Program?
Now that a fast, easy and reliable test for measuring the RUL of a lubricant is available, how can it be integrated into oil analysis and maintenance programs? First, it is important to evaluate the manner in which the more expensive RPVOT test is deployed. The use of RPVOT is typically limited to an annual, semiannual or quarterly analysis of large-volume systems, like turbine generators.
While the oil may be tested biweekly or monthly for viscosity, AN, particle count, etc., the RUL is tested only occasionally. Cost is the primary reason RPVOT is only occasionally conducted and typically on only the most critical systems. The RPVOT test usually costs $200 or more per sample to complete.
So with a less expensive and easier to use RUL technology, perhaps we could test RUL more frequently on large critical systems and expand its application to other machines that historically would not have been targeted, like hydraulic systems, gearboxes, etc.
The initial reaction for most people is that increasing the frequency of RUL testing on large-volume systems like steam turbines is a great idea, but the idea of applying RUL measurement to small-volume systems like gearboxes and hydraulic systems doesn’t make sense because the oil cost on these machines is too insignificant to warrant RUL testing.
One might say it is cheaper to simply change the oil. Such a conclusion fails to consider the picture in its entirety. The primary purpose and benefit associated with oil analysis is that it enables smart maintenance decisions. Because RUL enables managers to forecast the need for lubricant maintenance, it facilitates effective planning and scheduling.
Likewise, because RUL measurement occurs in the proactive domain, lubricant degradation can be effectively headed off at the pass, avoiding collateral damage to the oil and to the machine. While the cost of the lubricant itself may be insignificant, the numerous sources of potential collateral damage to the machine that are described below should be considered:
Corrosion
The oxidation process produces a number of by-products, including acids. Acid attacks component surfaces, causing pitting and surface degradation. In the presence of abrasives, a corrosion-abrasion cycle can erupt whereby corrosion produces easily abraded material that reveals easily corroded nascent metal in a cycle that exaggerates the rate of wear. Water, which is also produced as a by-product of oxidation and ingested from other sources, increases the corrosive potential of acid in oil.
Varnishing
Polymers and oxides are other by-products of the oxidation process. When varnish comes out of solution, it tends to condense onto component surfaces. When varnish collects on gravity-feed oil passageways, starvation can result. When varnish collects on the return line of a flow-controlled bearing, such as those found on a paper machine, the obstruction results in an increased pressure differential across the bearing, which stresses the seals and can cause leakage.
Varnish deposits on servo, proportional and other tight-clearance hydraulic valves result in sticking, which can slow or stop operation. Compounding the issue, particles tend to stick to the varnish in valves, which further exacerbates the sticking problem and causes abrasive wear. Varnish deposits that accumulate in the inner surfaces of tanks, pipes and hoses are chemically active and shorten the life of new oil added to the system during a top-up or change.
Loss of Lubricity
Oxidation affects the physical properties of the oil, altering its flow and lubrication characteristics. Likewise, sludge suspensions produced as a result of oil oxidation provide polar surfaces to which active additives like antiwear agents adsorb to the surface of the sludge, rendering them unavailable to protect the machine surfaces, which is their intended function.
Foaming and Air Entrainment
The moment the base oil begins to degrade, the interfacial tension properties of the oil start to decline. Interfacial tension (related to surface tension) is the physical property that describes the ease with which oil separates from insoluble materials such as air and water. Entrained air affects the oil’s compressibility (bulk modulus). Oil is a good lubricant because it provides excellent film strength due in large part to its incompressibility.
In fact, the viscosity of oil increases under load in the contact zone, the basis behind the elastohydrodynamic lubrication regime found in many rolling contact situations. Air, which by contrast is highly compressible, diminishes the strength of the oil film, which increases surface-to-surface contact and wear.
In hydraulic systems, entrained air produces sponginess and loss of precision control, again due to the air’s compressibility. Air is the primary cause of gaseous cavitation, which can erode surfaces upon which implosion forces are directed. Under pressure, compression heats the entrained air bubbles (adiabatic compression) to the point that the hot air bubbles thermally degrade, or coke, the oil molecules with which they come into contact.
Likewise, inside the air bubble, microdieseling occurs due to the presence of hydrocarbon vapors (usually the lighter fractions of the oil). Oil that readily entrains air oxidizes at a rapid rate due to the increase surface area contact between air (the source of oxygen) and the oil. An exaggerated state of air entrainment can result in foaming. Foam inhibits heat dissipation. It can overcome the tank or sump in which the oil is contained and leak on the floor, causing slippage or even fire hazards. It also rapidly oxidizes, dropping acid and sludge into the oil.
Poor Water Demulsibility
Like air entrainment and foam tendency, water demulsibility, or the ability of the oil to separate from water, is a function of interfacial tension, which begins to rapidly decline when the base oil begins to oxidize.
Water causes rust, increases the rate at which corrosion occurs, hydrolyzes additives and base oil components, produces dangerous flash vaporization and vaporous cavitation conditions, compromises elastohydrodynamic lubrication in rolling contacts (roller bearings, pitch line of gear teeth, etc.), causes hydrogen-induced wear (embrittlement and blistering), washes polar additives out of the oil and exacerbates air entrainment and foaming. It almost goes without saying that it is important to manage water contamination. Managing water separation properties is a major component of water contamination management. Base oil oxidation adversely affects fragile demulsibility properties.
Filter Plugging
Varnish deposits and sludge can cause rapid filter depletion (a big cost on some machines). Likewise, the entrainment of water due to poor demulsibility properties adversely influences the filterability of the oil. Filterability relates to the ease with which a lubricant passes through a mechanical filter media. The presence of entrained water has a significant adverse influence on filterability.
If the ability to more effectively plan and schedule lubricant maintenance is not sufficient motivation to consider more frequent RUL monitoring for large-volume systems and introducing it to smaller volume machines like gearboxes and hydraulics units, the desire to avoid the collateral damage caused by base oil degradation should be. Now it’s just a question of execution.
Building RUL Measurement into Your Used Oil Analysis Program
For the purpose of building an oil analysis strategy, RPVOT-based analysis will be referred to as RUL and LSV-based analysis as RUL screening. An appropriate strategy for employing RUL and RUL screening must be developed. The strategy should consider the machine, the operating environment and the application. To decide when to include RUL screening into an oil analysis test slate, divide your equipment into three groups:
-
Large-volume critical systems for which RPVOT testing is currently being performed on a routine basis;
-
Smaller volume systems for which RPVOT is not currently being performed, but where oil analysis is used to drive condition-based change decisions; and
-
Smaller volume systems for which oil change is based on a schedule and oil analysis is used to identify unusual instances of lubricant degradation and to facilitate associated root cause analysis.
For large-volume critical systems where RUL analysis is currently being performed on a quarterly, semiannual or annual basis, RUL screening should be built into the routine monthly or biweekly oil analysis test slate. If these systems are already being routinely sampled for wear metals, particle count, etc., it is sensible to add RUL screening into the test slate.
In the event that RUL screening reveals a potential problem, exception-based RPVOT-based RUL analysis should be performed to confirm the condition. Also, the periodic (quarterly, semiannual or annual) RPVOT-based RUL analysis should continue to be performed because it is the time-proven method. In time, this strategy may be revised, but for now it is sensible, does not add much cost to the program and adds a great deal of information and capability to the program.
Conventional tests for lubricant degradation such as AN, FTIR and viscosity should not be eliminated because they can reveal important problems unrelated to RUL, such as wrong oil addition.
For smaller systems where oil analysis is used to drive condition-based change decisions, RUL screening should be incorporated into the routine (biweekly, monthly, bimonthly or quarterly) oil analysis test slate.
Typically, the routine sample will include tests to detect abnormal wear, excessive contamination levels and lubricant degradation. As previously discussed, detecting lubricant degradation after the fact limits maintenance planning and scheduling options and can lead to undesirable collateral damage.
Adding RUL screening to the routine test slate does not eliminate conventional tests for lubricant degradation such as AN, FTIR and viscosity because once again they can reveal important problems unrelated to RUL, such as wrong oil addition.
For smaller systems where oil analysis is not employed to drive condition-based change decisions, RUL screening should not be pursued. For these machines, lubricant properties tests are useful only for determining abnormal operating condition and to facilitate root cause analysis.
Should the decision be made to transition a machine to condition-based changes due to a new strategy to periodically decontaminate the oil using offline filtration, if the machine’s lubricant has been upgraded to a synthetic or some other strategic imperative has driven the decision, RUL screening should be added to the routine oil analysis test slate.
The value of RUL screening relative to various machine types should be clear. Therefore, it is now important to know where to get the data. The instrumentation for performing LSV-based RUL measurements is suited to both on-site and lab-based applications. Plants and facilities with the scale, the desire and the means to perform on-site oil analysis will find the instruments for performing LSV to be reasonably priced and easy to use.
In addition to the acquisition price, the ongoing cost to purchase the test reagents must also be considered. Again, these are comparatively reasonable, particularly if RPVOT is the point of reference. If the decision is made not to acquire the instruments to perform LSV on-site, an oil analysis laboratory can be contacted.
If the laboratory is company-owned and exists to support the plant’s needs, it may be possible to encourage the lab manager to add the technology to the lab’s offering, particularly if the oil analysis program managers at the other facilities supported by the laboratory are also interested in the service. If oil analysis is contracted with a commercial oil analysis laboratory, the lab manager or lab owner should be contacted and told why this information is valuable.
Chances are that more and more laboratories will add LSV to their offering, particularly now that the method has been standardized by ASTM.
Conclusion
Once the strict domain of large-volume, critical lubrication systems, such as steam turbines, RUL measurement is now within reach for a larger cross-section of the machines in the plant. Due to the high cost of the RPVOT, its application has historically been limited to the periodic and infrequent assessment of large critical systems.
Newly standardized by ASTM, LSV enables users to obtain valuable lubricant RUL information on a frequent basis for a wide variety of equipment at a very low cost per sample. This enables lubrication professionals to proactively manage the life of the lubricant, schedule lubricant maintenance and avoid the negative consequences of base oil degradation. The future is here, it’s time to put the technology and technique to work!
References
- ASTM D6810-02 - Standard Test Method for Measurement of Hindered Phenolic Antioxidant Content in HL Turbine Oils by Linear Sweep Voltammetry. American Society of Testing and Materials International, West Conshohocken, Pa.
- ASTM D6971 - Standard Test Method for Measurement of Phenolic and Aromatic Amine Antioxidant Content in Nonzinc Turbine Oils by Linear Sweep Voltammetry. American Society of Testing and Materials International, West Conshohocken, Pa.
- Kauffman, R.E. (1994). Rapid Determination of Remaining Useful Lubricant Life. Handbook of Lubrication and Tribology, Volume III. E. Richard Booser, Editor. CRC Press, Boca Raton, Fla.
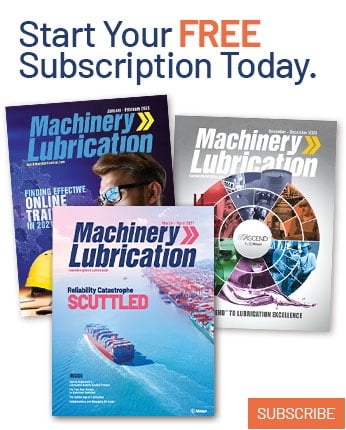