
Enclosed gear drives operate in three regimes of lubrication: full fluid film, mixed film and boundary lubrication conditions. The ideal regime of lubrication for enclosed gear drives is full fluid film lubrication, where the industrial gear lubricant’s viscosity is sufficient enough to provide separation of the mating surfaces of the gears and bearings.
Full fluid film is generally formed under conditions of light loads. As loads and pressures increase, mixed film lubrication exists, where the load is partially supported by the pressure developed in the lubricant film and partially by the contacting surfaces of the gears and bearings. Under mixed film conditions, the fluid film is pressurized but it is too thin to avoid contact of the highest asperities of opposing surfaces.
As loads and pressures further increase, this thin fluid film ruptures and boundary lubrication conditions exist. This is where metal-to-metal contact can occur.
Gear Wear Protection
In many industrial applications, enclosed gear drives are subjected to high loads, due either to their design or being used in applications for which they are underrated. All of these conditions can subject the industrial gear drive to operate under increased power densities. These higher power density loads are the direct result of increased horsepower and torque being applied to the gear drive.
These conditions can result in increased stress on the gears and bearings that can lead to accelerated wear. Therefore, it is important that the industrial gear lubricant being used has the ability to protect against abnormal gear and bearing wear, scoring, spalling and pitting, especially when high-load conditions are encountered.
This protection against wear is achieved by the use of antiwear and/or extreme pressure additive systems in the formulation of the industrial gear lubricant and, in some cases, by the addition of solid lubricants such as molybdenum disulfide, graphite, borates and oil soluble organomolybdenum compounds.
Extreme pressure and antiwear additives prevent metal-to-metal contact by adding film-forming compounds that protect the surface either by a physical absorption or a chemical reaction with the metal surface in order to form a low shear film at the point of contact.
Several tests measure an industrial gear lubricant’s antiwear and extreme pressure properties. Such test methods used to evaluate an industrial gear lubricant antiwear and extreme pressure properties include:
- Four Ball EP ASTM D2783
- Four Ball Wear Test ASTM D4172
- Falex Continuous Load ASTM D3233
- FZG ASTM D5182, DIN 51 354
- Timken EP Test ASTM D2783
The load wear index is an index of the ability of a lubricant to prevent wear at applied loads, while the weld point is the lowest applied load at which the sliding surfaces of four steel balls seize and weld together.
Four Ball EP ASTM D2783
In the ASTM D2783 Four Ball EP, steel balls of the same size and metallurgy are used (Figure 2).
Figure 2. Four Ball Wear Test
Three steel balls, which are immersed in the industrial gear lubricant being tested, are locked into a test cup. A fourth steel ball that is held in place in a rotating chuck is placed on top of the three steel balls locked in the test cup. The fourth steel ball is rotated at a speed of 1,770 rpm and subjected to a series of 10-second durations at increasing loads until welding of the steel balls occurs.
At the end of each 10-second test, the ball in the chuck is discarded and the other three balls are taken from the cup to examine the diameter of the wear scar. These wear scar diameters are used to calculate the load wear index. New steel balls are used with each load applied.
The loads are applied in a stepped series with the first load being 6 kilograms of force (kgsf). The loading series used is 6, 8, 10, 13, 16, 20, 24, 32, 40, 50, 63, 80, 100, 126, 160, 200, 250, 315, 400, 500, 620 and 800 kgsf. If welding does not occur at 800 kgsf, the lubricant is reported as having a weld point of +800 kgsf.
Four Ball Wear Test ASTM D4172
The Four Ball Wear Test ASTM D4172 evaluates an industrial gear lubricant’s wear-preventing properties in steel-on-steel sliding conditions. This test is not intended to predict wear characteristics with other metal combinations other than steel-on-steel and does not measure the extreme pressure characteristics of the industrial gear lubricant.
The Four Ball Wear Test ASTM D4172 utilizes the same test apparatus and set-up as the Four Ball Extreme Pressure Test ASTM D2783. The test is run at 1,200 rpm under a 40 kgf load at 167ºF (75ºC) for one hour. After the one-hour test period, the three stationary balls are washed and the wear scars are measured to the nearest 0.1 mm.
Another set of measurements across each scar, 90 degrees from the first, is then made. The average of the six measured scars in millimeters is reported as the average scar diameter.
The lower the scar diameter, the higher the level of wear protection the industrial gear lubricant will provide under sliding conditions. When comparing the capabilities of industrial gear lubricants, the results obtained in both the ASTM D2783 Four Ball EP and the ASTM D4172 Four Ball Wear Test should be considered. Some industrial gear lubricants that have good extreme pressure properties may not be effective in reducing wear rates at lower loads.1
Falex Continuous Load ASTM D3233
The Falex Continuous Load Test ASTM D3233 is used to measure the load-carrying properties of fluid-based lubricants (Figure 1).
Click Here to See Figure 1. Falex Continuous Load Test
This test method has been found to have some direct correlation to field service conditions, where high-load conditions are encountered. The test utilizes two procedures to evaluate the industrial gear lubricant’s load-carrying properties and its ability to protect against scuffing. Procedure A is known as the Falex Run-Up Test Method, while Procedure B is referred to as the Falex One-Step Test Method.
Both test procedures consist of running a rotating steel pin at 290 rpm driven by a 1/3 horsepower motor against two stationary V-blocks that are immersed in 60 ml of the industrial gear lubricant being tested. The load applied to the V-blocks against the rotating pin is applied through the use of a ratchet mechanism. In Procedure A, increasing loads starting at 300 pounds of force (lbf) (1,334.4 newtons) are applied continuously.
In Procedure B, the loads are applied at a starting point of 250 lbf (1,112 newtons) with the load being maintained for one-minute intervals at increasing loads. In both test methods, the test ends when the industrial gear lubricant being tested can no longer support the applied load, resulting in either breakage of the pin or seizure of the pin to the V-blocks.
The highest load that can be applied in this test is 4,500 lbf (20,016 newtons). An industrial gear lubricant that exhibits a 1,500 lbf (6,672 newtons) is considered to provide sufficient protection against gear wear and scuffing under heavily loaded conditions.
FZG ASTM D5182, DIN 51 354
The FZG ASTM D5182, DIN 51 354 is used to evaluate the antiwear, load-carrying and scuffing load capabilities of an industrial gear lubricant. The FZG gear set simulates a misaligned gear set operating in a bath of lubricant. The FZG gear set consists of two gear sets arranged in a four-square configuration driven by an electric motor delivering at least 7.4 horsepower at 1,440 rpm.
The test gear set is run in the industrial gear lubricant being tested, while increasing load stages (1 to 13) until scuffing or scoring of the gear teeth occur.
Each load stage runs for 15 minutes at a constant speed of 1,450 rpm at a test temperature of 194ºF (90°C) beginning at the fourth loading stage. The different load stages subject the industrial gear lubricant to contact pressures ranging from 21,178 psi (4,928 kg/m2) for Stage 1 to 289,000 psi (141,101.4 kg/m2) for Stage 13.
The test gears are examined initially and after each load stage for scuffing or scoring of the gear teeth. The stage at which more than 20 percent of the load-carrying flank area of the pinion is damaged by scratches and/or scuffing is considered to be the failure load. The higher the load stages, the better the industrial gear lubricant’s resistance to scuffing.
Timken EP Test ASTM D2783
The Timken EP Test ASTM D2783 provides a rapid method for measuring the abrasion resistance and the load-carrying capabilities of industrial gear lubricants. The test method is widely used for specification purposes and to differentiate industrial gear lubricants that have low, medium and high levels of extreme pressure characteristics. Any test results that are obtained by the use of this test method have been found not to correlate with results obtained during field service.
During the test, one gallon of the industrial gear lubricant being tested is placed into the test mechanism’s reservoir. The tester is operated with a Timken tapered roller cup rotating against a stationary hardened steel block at a rotating speed of 405.88 ±2.54 ft/min., which is equivalent to a speed of 800 rpm.
The industrial gear lubricant sample is brought to a test temperature of 100°F (38ºC), and applied to the test cup and block by gravity from the one-gallon reservoir and by a scavenging pump that returns the lubricant to the reservoir. Two determinations are made: the minimum load (score value) that will rupture the lubricant film between the rotating cup and the stationary block and cause abrasion; and the maximum load (OK load) at which the rotating cup will not rupture the lubricant film and cause abrasion between the rotating cup and the stationary block.
Fixed weights acting through a 10-to-1 ratio lever arm are applied by an automatic loading device in order to force the block into contact with the rotating test cup. Each load is applied for 10 minutes. At the end of each 10-minute run, the stationary test block is examined for scoring. Scoring is indicated when the edges of the wear scar becomes uneven. The OK load is the last load before scoring has occurred.2
An extreme pressure industrial gear lubricant is considered to have good extreme pressure properties if it has a Timken OK load of at least 60 pounds.
Demulsibility Characteristics
Industrial gear lubricants are frequently exposed to water from damaged coolers, lines, water spray used for cool down, and atmospheric moisture or steam sources. Contamination of the gear lubricant with water can cause poor lubrication, rust and corrosion and acceleration of the rate of oxidation of the gear lubricant.
An industrial gear lubricant must resist mixing and emulsification with water and have good water separating characteristics for quick and effective water removal from the system. An industrial gear lubricant’s ability to resist emulsification and separate readily from water is achieved through the use of demulsibility additives in the formulation of the industrial gear lubricants.
Demulsibility additives are chemicals that work in two stages to prevent emulsification and enhance water separation. First, they flocculate the water droplets into bubbles by forming a chemical bridge between water droplets. This allows the water droplets to also interact with the demulsifier’s molecules that are absorbed on the droplet’s sheath. Coalescence occurs in the second stage.
Once the chemical bridge has been formed, the demulsibility agent penetrates the emulsion film and replaces the emulsified water molecules to form an unstable film. Demulsifiers can also penetrate the emulsified film to form a passage through which water drains from one droplet to the other. Both of these mechanisms allow small, stable bubbles to combine into larger bubbles that drop to the bottom so they can be drained off.
Though demulsibility agents are essential chemicals used in the formulation of industrial gear lubricants, care must be taken in their use, especially if they are added to the industrial gear lubricant during field use. If used at improper additive treatment levels, they can have the reverse effect and act as emulsifiers. The test methods used to evaluate an industrial gear lubricant’s abilities to resist emulsification and to separate readily from moisture include:
- Water Separability Characteristics of Petroleum and Synthetic Fluids ASTM D1401
- Demulsibility Characteristics of Lubricating Oils ASTM D2711
Water Separability Characteristics of Petroleum and Synthetic Fluids ASTM D1401
The Water Separability Characteristics of Petroleum and Synthetic Fluids ASTM D1401 test determines the ability of lubricating fluids, such as rust and oxidation-inhibited industrial gear lubricant, hydraulic oils, and turbine oils that have viscosities of 28.8 to 90 cSt at 40ºC to separate from water.
In this test, 40 ml of distilled water and 40 ml of the fluid to be tested are measured into a clean 100 ml graduated cylinder and immersed into a bath at 130ºF (54ºC). The oil and water mixture is mixed for five minutes at 1,500 rpm through the use of a stirrer. At the end of five minutes of stirring, the sample is allowed to settle.
At five-minute intervals, the cylinder is lifted out of the bath and inspected for the amount of oil, water and emulsion layers. The amount of time it takes for complete separation of the water and oil layers is recorde d. If complete separation does not occur after 30 minutes, the volumes of oil, water and emulsion remaining are recorded. An industrial gear lubricant that has a reading of 40-40-0 (oil-water-emulsion) is considered to possess excellent water separating characteristics.
Demulsibility Characteristics of Lubricating Oils ASTM D2711
Like the ASTM D1401 test, the Demulsibility Characteristics of Lubricating Oils ASTM D2711 measures an industrial gear lubricant’s ability to separate from water. The Demulsibility Characteristics of Lubricating Oils ASTM D2711 test is primarily used to test medium and heavy viscosity (ISO 220 to ISO 1500) industrial gear lubricants’ ability to separate from water.
The test consists of mixing 405 ml of the industrial gear lubricant being tested with 45 ml of water at 180ºF (82ºC) for five minutes using a special 4500 rpm stirrer. After a five-hour settling period at 180ºF (82ºC), the amount of water separated from the emulsion is measured. At the end of the five-hour settling period, a 50 ml sample of oil is withdrawn by the use of a pipette from the center of the funnel, two inches below the surface of the oil-water mixture.
This sample is discharged into a centrifuge tube and centrifuged for 10 minutes to determine the amount of water present in the sample. This amount is reported as the percentage of water in oil.
After sampling, the funnel is removed from the bath and any free water that has separated is drawn off into a 50 ml graduated cylinder. The amount of water drawn off is reported as total millimeters of free water. Once the free water is removed from the funnel, the volume of fluid remaining is reduced to the 100 ml mark of the funnel by siphoning.
The remaining 100 ml of fluid is drained directly into a centrifuge tube and centrifuged for 10 to 15 minutes. The amount of water and emulsion separated by centrifuging is reported as milliliters of emulsion.
Rust and oxidation-inhibited gear oils that exhibit 36 to 45 ml of total water separation in this test are considered to show good demulsibility characteristics.
When this test is performed on extreme pressure gear lubricants, a modified procedure is used. This modified procedure consists of 90 ml of water, and 360 ml of water stirred at 2,500 rpm for five minutes at 180ºF (82ºC). After stirring for five minutes, the same procedure as described above is followed.
An extreme pressure gear lubricant is considered to possess good demulsibility characteristics if it exhibits 2 percent maximum water in oil; 80 ml of total free water minimum and 1 ml maximum of emulsion according to the U.S. Steel 224 and AGMA 9005 performance specifications and 1 percent maximum water in oil; 60 ml of total free water minimum and 2 ml maximum of emulsion per the AGMA 250.04 specification.
Rust and Corrosion Protection
There are many types of industrial gear drives that have parts made of copper, brass or bronze. An industrial gear lubricant that comes into contact with these parts must not be corrosive to these components. In addition to being noncorrosive to yellow metals, the industrial gear lubricant must also have the ability to prevent rusting of ferrous metal components.
Therefore, it is essential for the industrial gear lubricant to contain not only rust and corrosion-inhibiting additives, but to also co ntain an additive system that is not chemically aggressive to yellow metal containing parts. This aspect is particularly true for extreme pressure industrial gear lubricants. Extreme pressure additives are chemically active chemicals that form adherent surface films that transform to a ductile metal soap under high loads and contact temperatures.
Though they are essential and indispensable additive systems that provide protection against gear wear, care must be taken in the formulation of an industrial gear lubricant to prevent chemical aggressiveness to yellow metals. This is usually achieved by adding of metal deactivators and sulfur scavengers in the industrial gear lubricant’s formulation.
The test methods used to evaluate an industrial gear lubricant’s abilities to resist rust and corrosion include the:
- Copper Strip Corrosion Test ASTM D130
- Turbine Oil Rust Test ASTM D665
Copper Strip Corrosion Test ASTM D130
The Copper Strip Corrosion Test ASTM D130 is used to evaluate the corrosive tendencies of oils to copper containing materials.
Table 1. Copper Strip Rating System ASTM D130
In this test, special three-inch copper strips are cleaned, polished and immersed into a test tube containing the oil being evaluated. The test tube is held in a water bath for three hours at 212ºF (100ºC). At the end of the exposure period, the strip is removed and cleaned. The strips are compared to a specially prepared set of standardized reference strips and rated against these standards on a scale of Class 1 (slight tarnish) to Class 4 (heavy tarnish).
An industrial gear lubricant that exhibits a 1b classification in this test is considered to exhibit good resistance corrosion to yellow metals.
Turbine Oil Rust Test ASTM D6654
The Turbine Oil Rust Test ASTM D665 is designed to measure the ability of industrial oils to prevent rusting under conditions of water contamination.
The test consists of two parts. Part A uses distilled water, and Part B uses synthetic seawater. The test consists of stirring a mixture of 300 ml of the oil being tested with 30 ml of water, either distilled or sea water, at 140ºF (60ºC) for 24 hours. A special cylindrical steel test specimen made of #1018 cold-finished carbon steel is polished and then completely immersed in the test fluid. At the end of the 24-hour test period, the specimen is removed, washed with solvent and rated for rust.
To pass the ASTM D665 test, the specimen must be completely free from visible rust when examined without magnification under normal light.
Finally, an industrial gear lubricant’s rust and corrosion-inhibition properties can be further enhanced by the industrial gear lubricant’s ability to resist oxidation and thermal breakdown and its ability to resist emulsification.
Resistance Against Foaming
All industrial gear drives are exposed to airflow. The churning action of the gears and bearings through the industrial gear lubricant can cause air entrainment or foaming, especially in high-speed gear sets. If a gear lubricant begins to foam, that can result in the following:
- Increased operating temperatures
- Inadequate lubrication due to reduced oil flow, which can result in a reduction of the oil film between the mating surfaces of the gears and bearings
- Spongy and erratic operation
- Loss of the gear lubricant due to overflow
- Increased oxidation of the gear lubricant
- Erosion and cavitation
- Increased gear and bearing noise
The industrial gear lubricant’s ability to resist foaming and air entrainment is achieved through the use of antifoam agents in the formulation of the industrial gear lubricants.
Antifoam agents are surface-active chemicals that consist of either methyl silicone polymers or acrylate copolymers. They prevent foam by changing the surface tension of the air bubbles as they are formed. This change in surface tension causes air bubbles to either collapse or rupture. The addition of only five to 10 parts per million of antifoam agent in the formulation of the industrial gear lubricant is required for effective foam control.
Foaming Characteristics of Lubricating Oils Test ASTM D892
The Foaming Characteristics of Lubricating Oils ASTM D892 test method is used to determine the foaming tendency of lubricating oils.
The test method consists of three sequences performed at different temperatures. The industrial gear lubricant being evaluated is aerated by the use of a gas diffuser at the test temperature for that sequence with dry air at a flow rate of 95 ml/minute.
Before beginning the first sequence, the oil sample is heated to 120°F (49°C) and allowed to cool to 75°F (24°C). 190 ml of the sample is poured into a 1000 ml graduated cylinder and the cylinder is immersed into a water bath that is maintained at the test temperature for that sequence. The sample is aerated for five minutes and the amount of foam generated at five seconds after disconnecting the gas diffuser is reported.
At the end of a 10-minute settling time, the amount of foam left is recorded. The foam is collapsed and for the next two sequences, the same procedure is repeated except the test temperatures used are 200°F (93.5°C) for Sequence II and 75°F (24°C) for Sequence III.
In this test, AGMA 9005-D94 specifies that an industrial gear lubricant exhibit no greater than 75 ml of foam at the end of five minutes of aeration and no more than 10 ml of foam at the end of a 10-minute settling time.
References
- Noria Corporation. Best Practices for Machinery Lubrication Course Manual, Lubricant Properties and Tests.
- Lubrizol Corporation. Industrial Lubricant Test Booklet. p. H-20.
- Lubrizol Corporation. Industrial Lubricant Test Booklet. p. H-25.
- Lubrizol Corporation. Industrial Lubricant Test Booklet. p. H-10.
Editor’s Note:
Lawrence G. Ludwig, Jr. is chief chemist/technical director for Schaeffer Manufacturing in St. Louis, Missouri.
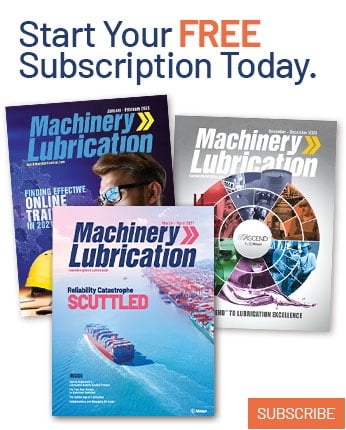