The most widely used laboratory methods for initial detection of abnormal levels of wear debris in used oils include elemental analysis, ferrous density analysis (DR, etc.), particle counting and patch testing.
For some users, because of the criticality of their machines, all of these screening tests for wear metals are integrated into the routine test slate. In such cases, when sampling is done correctly, it would be rare for the abnormal production of wear metals to go undetected.
However, when only one or two of these methods are routinely deployed, there is a distinct risk that an incipient (early stage) failure condition may be overlooked or dismissed as inconsequential.
For instance, most people familiar with used oil analysis understand the limitations of elemental spectroscopy (ICP, rotating disc electrode, etc.) with respect to quantifying actual levels of particulate suspensions in used oils.
Regardless of the type of spectrometer, particles larger than 10 microns are generally not measurable or are grossly understated in concentration.
There have been many reported cases where this limitation led to “missed saves” when the limitations of elemental analysis were not compensated by other large-particle techniques such as particle counting, ferrous density analysis and patch testing. The following are examples of how these methods, when used alone, can fail to detect incipient wear conditions:
-
Abrasive dust particles from a failed 15-micron filter missed by elemental spectrometers because the particles exceeded the size-specific detection limit of the instrument. A particle counter would have better detected the condition.
-
Flash-rusting of critical frictional surfaces missed by ferrous density instruments because red iron oxides are generally nonmagnetic and therefore not detectable. Instead, patch testing, particle counting and/or elemental analysis would likely have detected the abnormal corrosion condition.
-
Severe corrosion of bronze bushings from nitric acids in a natural gas engine missed by particle counting because the corrosion debris is too small and low in concentration to be detected. Direct reading ferrography also fails to detect these particles because of its limited sensitivity for small, nonferromagnetic particles. The condition would likely have been detected by elemental analysis.
- Wrongly classifying high particle counts of new gear oil as normal levels of undissolved additives. Use of a better sample preparation technique along with microscopic analysis would have confirmed that particles came from a botched flush job.
Occasionally, elemental data from used oil analysis will breach strategically placed alarms by large measure. Or, the reason for a large metallic debris field on a patch or filtergram cannot be satisfactorily explained.
Often, as in these cases, routine oil analysis data is insufficient in identifying the source and severity of problems without further analytical investigation. However, once an initial “exception” has been flagged, the shrewd oil analyst can deploy several companion tests to expand the analytical horizon.
The following discussion introduces the analysts to several such techniques that, when justified, can offer more clues and symptomatic information to complex wear conditions beyond what is generally provided by routine screening techniques with elemental analysis, particle counting and ferrous density analysis alone.
While some of the techniques discussed below require specialized analytical instruments and procedures, most are within reach of traditional used oil analysis laboratories. And where specialized requirements are prescribed, these tests (SEM, XRF, PIXE, etc.) can typically be easily outsourced to specialized labs.
The proposed analytical sequence is described below (Figure 1). The capital letters appearing in parentheses correspond to specific positions in the schematic, given for navigational purposes.
First - Separate Dissolved from Particulate Metals
The source of dissolved metals in used oil is distinctly different than particulate metals. While rotating disc electrode (spark-arc) and ICP emission spectroscopy offer little to no measurement sensitivity to larger wear particles, test results do not distinguish between soluble fractions and particulate metal fines. However, from a diagnostic and troubleshooting standpoint, the difference could be quite meaningful.
Begin by baselining the used oil by direct spectrometric measurement of wear metals in the oil sample (A) using a spark-arc or ICP spectrometer. For best results, a microwave acid-digestion procedure can be used to break down (solublize) large metallic particles first so that they too can be measured by the spectrometer.
Sulfated Ash Procedure
Another method to obtain an overall gravimetric indication of inorganic soluble and insoluble oil constituents is the common sulfated ash procedure (B) described in ASTM D 874-96 (note the interferences indicated in the procedure document).
Included in the sulfated ash result are dissolved and particulate wear metals, ash-producing solid contaminants (silica, etc.) and some new and decomposed organometallic additives (some AW additives, detergents, etc.). The test result does not distinguish between the metallic elements that comprise the ash.
Next, agitate the remaining sample and mix an aliquot 50/50 with reagent-grade kerosene or other suitable solvent. Transfer the mixture to an appropriate test tube and centrifuge2 (C). The insoluble wear metals, contaminants and coagulated (spent) additives will collect at the bottom.
Soluble metals will remain in suspension. Using a laboratory syringe, decant enough fluid from the top portion of the tube for additional analysis by elemental spectroscopy, sulfated ash and blotter spot testing.
After correction for the dilution by the kerosene, this elemental value represents the soluble metals (D) and the difference between this value and the baseline (A) is the apparent particulate metal concentration.
Again, the accuracy of (A) is improved if acid digestion is used. Soluble metals are from additives (both active and spent), some contaminants, some metal salts generated at AW/EP boundary contacts, and some nonferrous metals resulting from corrosion.
Optionally, by performing a sulfated ash test on the decanted fluid, an overall concentration of ash-producing soluble constituents (metals, inorganics, etc.) of the oil can be quantified (E).
When (E) is subtracted from (A), the difference is the insoluble (particulate), inorganic constituents of the oil. This value might include wear debris, particulate contaminants and some spent organometallic additives (ZDDP for instance).
Blotter Spot Test
Another interesting procedure that can be performed on the decanted fluid is the blotter spot test (F). Because oxides, dispersed carbon fines, resins and microorganisms have low specific gravity, it is possible that they may not separate by the centrifuge.
On the other hand, the presence of these borderline insoluble suspensions may reveal themselves in radial patterns and rings using the blotter spot procedure (paper chromatography) or planar radial chromatography. (ref. 2,3)
Next - Separate and Analyze the Nonferrous Particles
Transfer the remaining contents of the test tube, including the sediment to a small flat-bottom beaker or flask. Use a solvent such as kerosene to rinse out all the remaining sediment that occludes to the bottom of the tube.
Next, hold a powerful disc-shaped magnet directly underneath the beaker and swirl the mixture for a couple of minutes. This will magnetically deposit the ferrous debris (iron and steel) onto the beaker’s bottom surface (G) in the shape of a ring or wheel (we like to call this a ferrous wheel).
Without removing the magnet, quickly pass the liquid through a two-micron membrane (after tare-weighting) and rinse any remaining oil residue from the beaker through the membrane with kerosene. Dry the membrane in an oven and dispose of the effluent.
At this point, all of the nonferromagnetic particles should be on the membrane surface, while the ferrous particles should still be in the beaker. The following analyses can now be performed on the nonferrous debris:
Examine the Nonferrous Particles Microscopically
Using optical wear particle identification techniques (analytical ferrography), examine the physical characteristics of the particles on the membrane (size, shape, color, etc.) using a microscope (H).
Optionally, chemical microscopy, light effects (transmitted/reflected), heat treatments and impaction testing can be used to assist in the wear particle identification to determine source, mode of generation and severity (I). Nitrate membranes with a clarifying solution will be needed for effective use of bottom lighting. So too, thermal and chemically resistant glass fiber membranes would be required for heat treatments and chemical microscopy.
Gravimetrically Quantify the Nonferrous Concentration
Weigh the membrane and calculate the net concentration (J) of the nonferrous filtrate (report in milligrams per liter or ppm by weight). This can be trended routinely or, on exception, compared to benchmarked levels for both healthy and abnormal wear conditions.
Next, wash the membrane with pentane to dissolve organic material including friction polymers, oxides, additive floc, resins, sludge, etc. After drying, weigh the membrane again to determine the net concentration of the nonferrous, nonorganic debris (K).
The difference between (J) and (K) is the net concentration of insoluble organic matter relating to thermal, oxidative and other basestock/additive degradation products of the oil.
Identify and Quantify the Membrane Debris Elementally
Using X-ray fluorescence (XRF), proton-induced X-ray emission (PIXE) or other similar nondestructive methods, determine the cumulative elemental properties of the particles on the membrane (L).
Alternatively, transfer the particles to a suitable substrate and evaluate individual particles in the debris field (N) using a scanning electron microscope/energy dispersive X-ray (SEM/EDX).4 This is particularly important in identifying the probable metallurgy and source of suspect wear particles observed microscopically.
Particle Count by Resuspension
The particle size distribution of the nonferrous particles can be obtained using a standard resuspension method (M) (FPRC-G-S2).5 The procedure involves placing the membrane and nonferrous particles in a small beaker of superclean, low-viscosity oil (about 10 to 22 cSt).
The beaker is then placed in an ultrasonic bath for four minutes. The membrane should be carefully lifted using laboratory forceps, shaken in the oil several times and then removed (leaving the particles behind in the oil).
Afterward, the particle size distribution and ISO code can be obtained by an automatic particle counter using a suitable procedure, such as ISO 11500 calibrated to ISO 11171. The significance of the particle count is to assess the severity (stage of failure) of the wear condition.
Generally speaking, as the wear condition increases in severity, more and more particles are generated (per unit time) and the particles become larger. Hence, the remaining useful life of the machine can be roughly estimated using particle size and count with the assistance of the resuspension method if there is sufficient historical data.
Finally - Separate and Analyze the Ferrous Particles
The ferrous particles remain in the beaker, pinned down by the magnet (ferrous wheel). After removing the magnet (G), add a small amount of solvent such as reagent-grade kerosene to the beaker and swirl the fluid around.
Next, transfer the solvent through a 0.2-micron membrane (after tare-weighting) and rinse any remaining oil residue through the membrane. The ferrous particles are now on the membrane’s surface. The following analytical procedures can be used to examine the ferrous particles:
Examine the Ferrous Particles Microscopically
Using optical wear particle identification techniques, examine the physical characteristics of the ferrous particles on the membrane (size, shape, color, etc.) using a microscope (O). Light effects (transmitted/reflected) and heat treatments can be used to assist in the wear particle identification to determine source, mode of generation and severity (P).
Heat treatment is particularly good at distinguishing between cast iron, low-alloy steels and high-alloy steels. Same as before, the heat treatment procedure will require a thermally compatible glass-fiber membrane.
Gravimetrically Quantify the Ferrous Debris Concentration
Weigh the membrane and calculate the net concentration (Q) of the insoluble ferrous debris (report in milligrams per liter or ppm by weight). This can be trended routinely or, on exception, compared to benchmarked levels for both healthy and abnormal wear conditions.
Determine the Particle Count Using the Particle Resuspension Method
As previously described, the particle count can be obtained on just the ferrous particles using the resuspension method (R). This information can be helpful in estimating the remaining useful life of critical wear generated from iron and steel surfaces.
Ferrous Density Analysis
Besides gravimetric analysis, the concentration of the ferrous debris on the membrane can be quantified directly (S) using a ferrous density instrument sold by suppliers such as CSI and Kittiwake. The device, as typically used, measures the magnetic flux of wear particles in oil samples.
However, because the ferrous particles are already deposited on the membrane, the membrane can be placed directly in the receptacle instead of the sample of oil, as is typically done.
An indexed magnetic flux reading can then be obtained and trended. Alternatively, the combined influence of ferrous density and ferrous particle size can be obtained using the direct reading ferrograph sold by Predict and the ferrous particle counter (fCA) sold by Entek-Rockwell.
Elemental Analysis of Individual Ferrous Particles
The ferrous particles can be transferred to a suitable substrate and evaluated elementally (T) using a scanning electron microscope/energy dispersive X-ray (SEM/EDX) as previously described. This is particularly important in identifying the probable particle metallurgy and source of suspect wear particles observed microscopically.
Because steels have varying concentrations of nickel, chromium, manganese, molybdenum and silicon, these elements can be used to help fingerprint specific wear surfaces.
Examples of How This Analytical Scheme Can Be Used
Case No. 1
A main-line helicopter gearbox lube is showing elevated ferrous wear metal trends. However, because so many frictional surfaces share the common lubricant (gears, bearings, seals, shaft, housing, spacers, etc.), identifying the source and severity of the wear condition is indistinct.
However, by following the analytical scheme, it was found that all of the wear metals were essentially insoluble and ferromagnetic (O).
By analyzing specific particles in the debris field elementally using SEM/EDX (T), it was discovered that some were nickel-rich while others were high in chromium. Comparing this to the metallurgical information on the gear components, the source of the wear points to a tapered roller bearing.
The bearing raceways are made from steel alloys of 2.5 percent Ni and 1.4 percent chromium. The roller, however, is comprised of steel specified at 12 to 14 percent Cr.
Case No. 2
A large, two-cycle locomotive diesel engine is showing sharply rising copper and iron trends. All other oil analysis results appear normal. The source of the increasing copper and iron levels needs to be found.
By following the analytical scheme, it was discovered that the copper was soluble (D) and the iron was rust (H). This pointed to cooler core leaching (source of soluble copper) and water-induced corrosion of iron and steel surfaces resulting from the coolant leak.
Case No. 3
A temperature probe in a thrust bearing pad on a centrifugal compressor is showing sharply increased temperature. Elemental analysis from routine used oil testing shows only a slight rise in lead and tin but a sharp jump in particle count.
This raises the question of whether there is impending bearing failure and if the Babbitt debris from thrust pad wear is being missed by the spectrometric results (particles too large).
Here the analyst will want to see the particles (H), determine their elemental constituents (L), and, if the wear metals are indeed Babbitt, estimate wear severity by particle resuspension. Large Babbitt particles were discovered and, after inspection, the bearing was found to be severely impaired.
Case No. 4
There is glitter-like wear debris observed on the filter paper during a recent filter change on a hydraulic control system deployed in a tower crane. The source, cause and severity of the problem are of critical concern because of safety implications. Because the sample is filter media and not oil, the centrifugal separation (C) is skipped.
The analytical scheme is picked up at the magnetic separation (G). The debris was found to be chrome-rich (T) ferromagnetic platelets. This prompted an inspection of the actuators, which discovered a heavily chipped, chrome-plated cylinder rod.
Summary
There are many routine oil analysis tests for detecting (screening) abnormal wear metals in used oils. When such exceptions are encountered, it is frequently important that an efficient analytical process be followed to assess the source, cause and severity. This article presents several useful schemes to better gain diagnostic insight and precision in troubleshooting anomalous wear conditions.
References
1. Swanson, S. A. and R. T. Alexander. “Quantitative Filter Debris Analysis (QFDA): Implementation on Rotating Disc Electrode Atomic Emission Spectrometer (RDE-AES).” JOAP International Condition Monitoring Conference, November 1994.
2. Fitch, J. C. “Using Oil Analysis to Control Varnish and Sludge.” Practicing Oil Analysis magazine, May-June 1999.
3. Herguth, William R. “Spotting Oil Changes Using Radial Planar Chromatography.” Practicing Oil Analysis magazine, July-August 2000.
4. Luckhurst, Terry. “Scanning Electron Microscopy for Wear Particle Identification.” Practicing Oil Analysis magazine, September-October 1999.
5. Fitch, E. C. Fluid Contamination Control, FES, Inc., 1988.
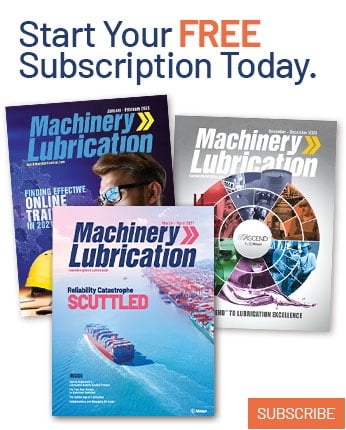