
Helical gears are used to reduce the speed of centrifugal compressors and turbines to match nominal motor and generator speeds. Proper lubrication and cooling are critical to the successful operation of these gears. When gears mesh, the tooth surfaces roll and slide against each other (Figures 1 and 2).
Figure 1. Helical Gear Terminology
Figure 2. Direction of Sliding
This meshing creates enormous contact and shear stresses. Oil is used to lubricate the mating gear teeth and prevent scuffing, wear and pitting damage to gear tooth surfaces due to metal-to-metal contact.
Oil viscosity increases with pressure; therefore oil can separate the mating gear teeth despite enormous stress. Oil is also used to cool the gear teeth and prevent excessive tooth temperatures and overheating of the oil.
This article presents various issues associated with proper lubrication and cooling requirements of high-speed helical gears. High-speed helical gears are defined as those gears that operate pitch line velocities greater than 120 m/s.
Gear Surface Distress
The mating surfaces of gear teeth are not perfectly smooth. The surfaces are full of small asperities, such as machining marks. This is referred to as surface roughness.
If the oil film is thick enough, the asperities from the mating gear teeth do not contact one another. Based on elastohydrodynamic lubrication (EHL) theory, lubrication falls into one of three regimes. In Regimes 1 and 2 there is some contact between the mating gear teeth.
Most high-speed gears fall into Regime 3, in which full EHL oil film develops and the asperities in the tooth surfaces do not contact one another.
Gear tooth distress includes scuffing, wear and surface fatigue (micropitting and macropitting). ANSI/AGMA 1010-E95 (1995) provides descriptions and pictures of these types of damage.
Scuffing
Scuffing is damage to a tooth surface due to welding and tearing of the tooth surface by the flank of the mating tooth. It is characterized as radial scratch lines (Figure 3). Scuffing occurs when the oil film thickness is less than the composite roughness of the pinion and gear and metal-to-metal contact occurs.
Figure 3. Scuffing (Courtesy of ANSI/AGMA 1010-E95)
Mild (Left), Severe (Right)
According to AGMA 925-A03 (2003), “The basic mechanism of scuffing is caused by intense frictional heat generated by a combination of high sliding velocity and high contact stress.”
Scuffing will normally not start at the pitch line because the sliding velocity is zero. Thus, scuffing generally starts in either the top or bottom half, or root, of the teeth. The contact temperature is equal to the flash temperature plus the tooth temperature before entering the mesh. AGMA 925-A03 (2003) states:
“The flash temperature is the calculated increase in gear tooth surface temperature at a given point along the line of action resulting from the combined effects of gear tooth geometry, load, friction, velocity and material properties during operation.”
Several sources can cause higher tooth temperatures at high pitch line speed. According to AGMA 925-A03 (2003), “For pitch line velocities above 80 m/s, churning loss, expulsion of oil between meshing teeth and windage loss become important heat sources that must be considered.”
The other factor is how effectively the oil spray cools the teeth. When spraying the gear mesh, oil must cover the tooth surfaces for a slight time interval before being flung off. At high pitch line speeds, the teeth may be moving so fast relative to the oil velocity that not all the teeth get covered with oil.
Wear
Wear is defined as the removal or displacement of metal from gear tooth surfaces. Damage due to scuffing or pitting is not normally considered wear. Wear reduces tooth thickness and can change the contour of the teeth.
Wear can result from mechanical, chemical or electrical action. For well-designed gears that operate in lubrication Regime 3, the rate of wear should be so slight that the original machining marks should still be visible after a year or more of service.
This low rate of wear also assumes that the supply oil is well-filtered and that there is no abrasive wear due to hard particles suspended in the oil.
Micropitting
Micropitting is a high rolling contact fatigue incident that occurs in the Hertzian contact band area. It is a function of combined rolling and sliding velocities, load, temperature, specific film thickness and the lubricant itself. AGMA 925-A03 (2003) states: the pits are typically 10 to 20 µm deep by about 25 to 100 µm long and 10 to 20 µm wide.
Because of the size of the pits, micropitting can be difficult to see. If micropitting progresses far enough, the micropits will coalesce and the surface will take on a dull, matte appearance (Figure 4).
Figure 4. Micropitting
(Courtesy of ANSI/AGMA 1010-E95)
Micropitting can lead to a reduction in gear tooth accuracy, which increases gear tooth loading, vibration and noise. Micropitting can lead to macropitting and gear tooth breakage.
There is a basic lack of understanding of the mechanism of micropitting. One theory claims that micropitting starts when the asperities on gear tooth surfaces carry a significant portion of the load. These asperities then deform, which produces local residual tensile stresses.
The cyclical loading is then high enough to cause local fatigue cracks that take on the form of small pits. Thus, surface roughness is a big factor in the risk of micropitting. According to AGMA 925-A03 (2003), micropitting was eliminated in some cases when the gear tooth surfaces were finished to a mirror-like finish.
Micropitting can occur anywhere on the gear tooth surface. However, according to researchers Cardis and Webster, it generally starts in areas associated with high sliding velocity, which is in the bottom or top of the tooth profile, not at the pitch line where the sliding velocity is zero.
Lubricants also play a key aspect in the risk of micropitting. Studies completed by Cardis and Webster have shown that micropitting is more apt to occur in gears that use oils with antiscuffing additives.
Also, micropitting resistance tends to decrease with higher gear tooth temperatures, but it has been reported that other additives actually improve micropitting resistance at higher temperatures.
Macropitting
Macropitting is also a surface fatigue phenomenon. These pits are typically on the order of 0.5 to 1.0 mm in diameter and are large enough to be seen by the unaided eye (Figure 5).
Figure 4. Micropitting
(Courtesy of ANSI/AGMA 1010-E95)
Macropits typically occur if there are high asperities and metal-to-metal contact occurs between meshing teeth. However, in high-speed gears, the surface finish is typically very smooth and the oil film is thick enough to prevent metal-to-metal contact.
In these cases, the cause of the macropits is generally an inclusion or small void in the material that provides the initiation point for the crack, and the subsurface shear stresses propagate the crack.
Thermal Problems
Another purpose of lubricating oils is to cool the gear tooth surfaces. Testing by Martinaglia showed that high-speed helical gear teeth could distort due to nonuniform temperature distribution along the face width of the gearing. In many cases, the working flanks of the pinion and gear have to be modified to account for this distortion.
At very high pitch line velocities, problems can also occur with overheating of the air/oil mixture trapped in the gear mesh. Varnish and carbon deposits can also form on gear tooth surfaces. These deposits close up the backlash and cause local overloading and gear tooth failure.
Gear Spray Arrangements
Oil is typically sprayed into the gear mesh from a spray bar. The spray bar extends across the width of the gears and is fitted with small nozzles that are used to create small oil jets that spray oil into the mesh. Based on a survey of several gear manufacturers, there is no universal agreement on whether oil should be sprayed into the in-mesh, the out-mesh, or both.
It depends on the experience of the gear manufacturer. It is important that the gear teeth are wetted with oil for proper lubrication and that enough oil gets into the tooth spaces for sufficient cooling.
Some gearbox manufacturers use special baffles to reduce windage losses inside gearboxes. Air inside the gearbox is accelerated because of the high rotational speed of the gears.
The energy that it takes to accelerate the air is a loss. By fitting plates close to the sides of the gears, the amount of air that is accelerated is limited and this loss is reduced. These plates can also be used as shields to prevent oil that is squeezed out of bearings from hitting the gears, causing acceleration, which would result in additional loss.
Finally, other gear manufacturers use a false bottom in the gearbox. This is typically a perforated plate that is fitted between the gears and the bottom of the gearbox. It is used to help the oil drain by preventing the oil in the bottom of the gearbox from being lifted back up and reaccelerated.
Special Designs
Greiner and Langenbeck performed tests with separate lubrication and cooling oil supply to observe the influence of this arrangement on gear temperatures (scuffing load capacity) and on efficiency.
One set of nozzles was used to spray either the in-mesh or out-mesh for lubrication and another set of nozzles was used to spray the gear webs for cooling. Temperature, power loss and wear measurements were taken for a multitude of spray arrangements, oil flows and flow splits.
Based on the testing, the separation of lubrication and cooling oil supply led to a 60 percent reduction in oil flow (compared with the original recommended oil flows), lower gear tooth temperatures and a slight increase in efficiency.
One company has a patented a gearbox that operates in a vacuum and virtually eliminates windage losses. This invention has reportedly lowered the gearbox power loss by up to 50 percent in comparison to conventional gearboxes.
One documented case was a comparison of two 90 MW gearboxes: one with conventional gearing and the other with the vacuum gearbox. The calculated losses in the conventional gearbox at full load were 1,407 kw, while the losses in the vacuum gearbox were measured at 628 kw. This is a difference of 779 kw, or about one percent of the rated power.
Several companies also manufacture high-speed helical gears with special axial grooves (Figure 6).
Figure 6. Axial Grooves for High-speed Gearing
These grooves provide a path for the hot air/oil mixture to escape before overheating and allow fresh, cool oil to be supplied to the gear mesh at an intermediate point in the mesh. Typically, these grooves are added on the bull gear and sometimes on the bull gear and the pinion. Although Figure 6 shows only a single groove, other designs may have more.
Various high-speed gearboxes are also equipped with a special high-pressure/high-velocity-type spray arrangement that is fitted close to the gear mesh (Figure 7).
Figure 7. High-pressure Gear Spray Arrangement
As stated above, it is important for proper cooling for the oil to cover the tooth surfaces for a slight time interval before being flung off. At high pitch line speeds, higher velocity oil may be needed to get sufficient cooling oil into the tooth spaces before being stuck by the next tooth and flung out of the gear mesh. Generally, more oil is required for cooling than for lubrication.
So while there may be enough oil on the gear tooth surfaces for lubrication, there might not be enough for cooling.
There are many good sources available to end users to assist in the evaluation of high-speed helical gear designs. Although lubrication and cooling problems are rare, they can be difficult to solve. When evaluating high-speed helical gears, it is important to understand the manufacturer’s experience. Lubrication and cooling are complex issues and a number of parameters must be considered.
Editor’s Note:
Reproduced with permission of the Turbomachinery Laboratory (http://turbolab.tamu.edu). From Proceedings of the Thirty-third Turbomachinery Symposium, Turbomachinery Laboratory, Texas A&M University, College Station, Texas, p. 41-48, Copyright 2004.
References
-
AGMA 925-A03. “Effect of Lubrication on Gear Surface Distress.” First Edition, AGMA, Alexandria, Virginia, 2003.
-
ANSI /AGMA 1010-E95. “Appearance of Gear Teeth – Terminology of Wear and Failure.” First Edition, AGMA, Alexandria, Virginia, 1995.
-
Cardis, A. and Webster, M. “Gear Oil Micropitting Evaluation.” Gear Technology, September/October 2000, p. 30-35.
-
Dudley , D. Handbook of Practical Gear Design. New York, McGraw-Hill Book Company, 1984.
-
Greiner, J. and Langenbeck, K. “Separation of Lubrication and Cooling in Oil-Jet Lubricated Gears.” AGMA Paper Number 91 FTM 13, 1991.
-
Martinaglia, L. “Thermal Behavior of High-speed Gears and Tooth Corrections for Such Gears.” ASME/AGMA International Symposium on Gears and Transmissions, San Francisco, California, 1972.
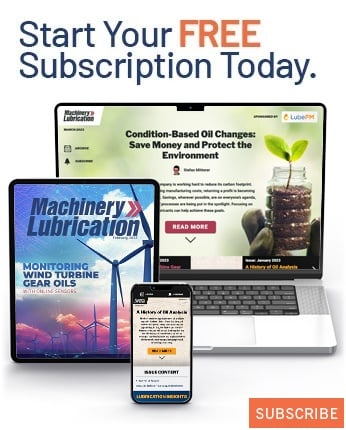