The Why and How of Oxidation Testing
It is widely understood that oxidation is the primary mechanism of lubricant degradation. It is also widely known that oxidized oil can’t effectively lubricate machines; oil analysis tests like Total Acid Number and FTIR-Oxidation can reveal abnormal oxidation. Less widely understood is how these tests monitor the onset and propagation of oxidation, and the significance of the results.
It is important for lubrication and oil analysis technologists to understand how lubricant oxidation is measured, and how these tests can best be applied to monitor the lubricant’s performance. A greater understanding of lubrication oxidation will enable technologists to make informed decisions to ensure lubrication excellence.
The Chemistry of Oil Oxidation
The fundamental key to understanding lubricant oxidation is having basic knowledge of the chemistry of lubricants and their degradation. The chemistry of lubricants is simply a specialized sub-set of organic chemistry. Organic chemistry is the study of carbon-based compounds and their reactions. Lubricant base oils, with a few exceptions, are classified as organic compounds because they are primarily hydrocarbons. Base-oil oxidation is an example of the many possible organic reactions that can occur in a lubricant. To fully understand the oxidation of lubricants, we first consider the generic reaction of hydrocarbon oxidation.
A hydrocarbon is a compound containing carbon and hydrogen. Although there are an infinite number of variations and complexities in the final compounds, a basic hydrocarbon structure can be represented in Figure 1.
In Figure 1, the black spheres represent carbon atoms, and the smaller white spheres represent hydrogen atoms. The links between the carbon and hydrogen atoms represent bonds that attract and hold the atoms together. This bonded structure is referred to as a compound. The number of carbons and the type of bonding present characterize a hydrocarbon compound. The compound represented in Figure 1 is n-Heptane, a common liquid hydrocarbon used as a reagent in some oil analysis tests.
Common lubricants are made up of compounds with longer chains and multiple bonding configurations. When describing the chemistry of lubricants and their reactions, it will usually suffice to show just the end of the molecule, or some part of a chain with the letter R representing an undefined chain of carbons, hydrogens and other atoms (see Figure 2). For the purposes of studying the oxidative reaction of the compound, the R is unimportant, since it does not factor into the reaction.
The most important chemical reaction of a hydrocarbon, for a lubrication engineer or analyst, is oxidation. Oxidation of hydrocarbons is commonly referred to as combustion or “burning.” When one burns paper, wood, natural gas or fuel oil, for example, hydrocarbons are oxidized. In a well-designed burner, propane gas undergoes complete combustion.
This means that all carbons are completely oxidized; all hydrogens bonded to carbons are replaced by oxygens, therefore producing CO2 and H2O. The reaction is illustrated in Figure 3 where five oxygen molecules (O2) completely oxidize the illustrated hydrocarbon to produce three carbon dioxide molecules and four water molecules.
Complete combustion requires relatively high temperatures, a pure fuel source and an ample supply of oxygen. Most combustion reactions found in nature, however, are not complete, and result in various other products in addition to CO2 and H2O, as illustrated above. Incomplete combustion of the hydrocarbon chain of a lubricant can produce carboxylic acid (Figure 4) and other impurities.
The double-link between the carbon atom and the top oxygen atom represents a double bond. This reaction illustrated in Figure 4 occurred at the end of a chain. Oxidation can also occur in the middle of the chain, splitting the hydrocarbon into two Carboxylic Acids as illustrated in Figure 5. In this case R represents some undefined chain and R1 represents a different chain but are unimportant because they are not involved in the reaction.
The Effect of Oxidation on Lubricant Properties
The most important reaction on lubricant properties is when a single chain is broken down into two smaller chains. This is when the chemical reaction of oxidation begins to transform the physical world of lubrication. There is no noticeable difference once this reaction starts to lubricate a bearing. However, a significant change can be observed when this oxidation reaction has occurred on a large percentage of the molecules in that reservoir.
By breaking down the chain, the ability of that molecule to carry the load between two moving solid surfaces has been altered. Additionally, the new shape of the molecules will cause them to interact in a different physical manner with the normal lubricant molecules, and can result in a greater than normal viscous heating. Imagine a room covered with a single layer of uniform marbles and then lay a flat board on top of them. The board will slide around fairly easily because the marbles act like ball bearings.
But if you were to add a few ping-pong balls to the room, the board would not move as smoothly; there is increased friction caused by the presence of the Ping-Pong balls. The increases in viscosity that are seen as a result of oxidation can be explained by pointing to the polymerization of products such as sludge. However, with only the mechanism of oxidative cleaving of hydrocarbon chains, there can be a net effect of increased viscosity due to the strained interaction of non-homogeneous compounds in the lubricant. Just like Ping-Pong balls interfere with the relative motion of a board moving across marbles, non-homogenous molecules impede the relative motion of fluid.
Where previously the molecules in the oil were similar in size and shape and flowed fairly freely among each other, the new compounds created from oxidation are quite different and introduce new forces of interaction between the molecules. For instance, carboxylic acid compounds can be highly associated, meaning that there is an attraction between the molecules, causing them to behave as a larger molecule. (See Figure 6)
his associated molecule is known as a dimer, and is suggested in lubricants by the occurrence of the carboxylic acid carbonyl peak on the infrared spectrum. In oxidized lubricants, the peak is seen at 1710 cm-1, indicative of an associated carbonyl, instead of the unassociated peak at 1760 cm-1. The net effect is a greater resistance to flow at the molecular level, which can have the bulk effect of a viscosity increase in the lubricating fluid.
Oil Analysis Indications of Lubricant Oxidation
The oil produces numerous warning signals indicating oxidation that can be detected with oil analysis. Below is a review of the most common warning signals.
Total Acid Number (TAN) - As previously discussed, organic acids are produced during oxidation. These acids are detectable as an increase in the TAN number which quantifies acid concentration by measuring the volume of an alkaline (potassium hydroxide) reagent that is required to neutralize the acid in the oil. The TAN test doesn’t discriminate acids generated by oxidation from those that are ingested as contaminants from the process. Also, some additives like anti-wear, extreme pressure and some rust inhibitors, are acidic. They produce a high initial TAN that can diminish as the additive is depleted.
Viscosity - As carboxylic acid byproducts of oxidation dimerize (or associate), the median density of the molecules increases. This results in increased viscosity. Viscosity can be measured using kinematic or absolute methods.
Fourier Transform Infrared (FTIR) Spectroscopy - This technique involves passing a beam of infrared energy through a sample of oil. Different molecules absorb infrared energy at different frequencies. As the oil oxidizes, hydrocarbons transition to aldehydes, ketones, alcohols and carboxylic acids that are detectable by the FTIR spectrometer as new compounds.
Darkening Color - For various reasons, as the oil oxidizes, its color tends to darken. While dark oil is not always oxidized and degraded, it is one possible indication.
Foul Odor - As the oil oxidizes, it often assumes a putrid odor. If the base-oil contains sulfur components, or the oil is equipped with a sulfur-containing additive, the odor may resemble that of a rotten egg.
Conclusions
Lubricant oxidation is serious business. Not only is the lubricant’s performance diminished, the acids produced can increase corrosive component wear. It is important to understand the process and root causes of oxidation and the warning signals generated by oil analysis. Managing and measuring lubricant oxidation is the key to the pursuit of lubrication excellence.
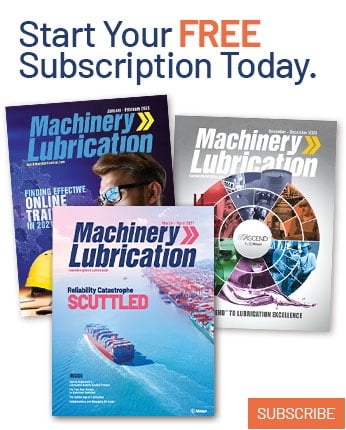