Introduction
We have known for decades that clean oil will improve system reliability and uptime, as well as prolong component and oil life in service [1]. This is especially top of mind for wind turbine owners and operators. Oil analysis is used to predict failure and hinder un-scheduled downtime. However, the oil analysis mainly shows the status at the specific time of the sample, and it is difficult to ensure the sample is completely representative [2]. The oil cleanliness in a wind turbine gearbox (WTG) is hugely affected by load, idle or standstill, and only sampling every six months makes trending even more inaccurate.
This is where online sensors show their value: they accurately monitor many times per hour and give immediate access to trending data. This article will evaluate oil cleanliness and the state of oil degradation/quality on 10 wind turbine gearboxes using online sensors.
Figure 1: Near-shore wind turbines
Monitoring Oil Using Online Sensors
Online oil sensors give real-time data and help you predict a bad trend, which could lead to a serious situation. The best online condition monitoring systems consider oil degradation, water content and particle count as well as machine load. In this paper, we will focus on particle counts and oil degradation/quality measured by resistivity since water issues are quite rare in wind turbine gear oil.
Obtaining the best possible installation point for the online sensors is vital to get the most representative data. Tests have shown that the offline/kidney loop oil filter circuit is ideal for online analysis due to the continuous homogeneous flow and suction from the bottom of the oil reservoir [3]. Optimum conditions are thereby present for the evaluation of particles, water and oil degradation.
Oil Degradation Sensors
The oil quality or state of degradation can be evaluated using sensors that measure resistivity, which correlates to oil aging by oxidation, acidity and water content. Resistivity is well-known in the power industry, where transformer oils have been monitored by resistivity for more than 50 years [4].
Figure 2: Oil Quality sensor.
A reduction in resistivity indicates the oil is degraded or contaminated. Resistivity sensors can thus be used to assess the oil quality/degradation and recommend actions such as sweetening, filtration or a full oil change.
In gearboxes, degraded oil will often result in varnish and increased viscosity, which create problems like increased friction, poor cooling, etc. In addition, the oil may be difficult to pump during cold start-ups, which may lead to cavitation or even starvation. Oil degradation will reduce the oil’s in-service life, which will result in premature oil changes, increased gearbox wear and even risk of a complete failure.
The photo below shows progressive states of oil degradation [5], which can be detected by means of reduced resistivity (online Oil Quality sensor).
Figure 3: States of degraded oil.
Online Particle Counting
Particles will be created during the operation of any machine, influenced by load, rotational speed, oil temperature, oil additives, etc. [6]. But if the oil is kept mostly free from contaminants, wear will be reduced to a minimum. Furthermore, it is much easier to discover an abnormal wear trend when the oil cleanliness is good, compared to seeing an increase in particle counts in a very contaminated oil.
Benefits of Online Particle Counting
• Early warning: if the trend increases, a worn component can be replaced before larger issues arise, e.g., replacing a ball bearing before a total breakdown of the gearbox.
• Oil analysis and maintenance can be scheduled according to the online data, e.g., indicating a bad wear trend, which requires an in-depth oil analysis or onsite investigation.
• Instant data access and visible trends can support more precise decision-making in case of overhauls, as well as improved maintenance practices and intervals.
Trending Data Using a Web-Based User Interface
The data in this study is collected using C.C.JENSEN’s cloud solution (CJC® T2render) [7], which receives data from the CJC® Condition Monitoring Unit (CMU) [8]. The CMU can incorporate multiple sensors, but in this study, we will focus on particle counts according to ISO 4406 as well as resistivity, indicating the oil quality and state of degradation.
Figure 4: Condition Monitoring Unit with sensors,
connected to the CJC® offline filter, which is
installed as a kidney loop on the gearbox.
From the sensors, the encrypted data are sent via a secure connection (4G, Wi-Fi or Ethernet) to a cloud-based solution for further analysis. The data can be provided directly to a surveillance system (SCADA system) or followed on a web-based user interface.
Alarms are sent to the operator by email or text message when pre-set limits are surpassed or when the individual system oil and equipment trend varies from normal operation. This reduces the complex and time-consuming interpretation of data from individual sensors from influencing each other.
Figure 5: The web-based graphical user.
Case Study — Monitoring Particles and Oil Quality/Degradation on 10 Wind Turbine Gearboxes
This study looks at data taken from 10 WTGs installed in Europe, North America and South America, with rated power from 0.9 MW to 3 MW and a mix of on-shore, near-shore and off-shore installations.
Overview:
• 4 x Siemens, 3 MW (off-shore)
• 2 x Siemens, 2.3 MW (near-shore)
• 1 x Vestas V90, 2 MW (on-shore)
• 1 x GE, 1.6 MW (on-shore)
• 2 x NEG Micon, 0.9 MW (on-shore)
The 10 gearboxes were all fitted with a CJC® 3-micron offline/kidney loop oil filter for maintaining gear oil cleanliness. Some of the WTGs had the 3-micron offline filter (OEM fitted), and some had the filter retrofitted later in the WTG’s life.
Figure 6: A microscope photo of abrasive wear.
During the operation of a gearbox, any changes in load, start/stop, temperature, wind gusts or other stress factors will create particles visible to the online particle counter. Smaller particles, up to 10 microns in size, are seen during normal abrasive wear (4 and 6-micron counts in the ISO 4406 codes), while particles larger than 10 microns indicate severe wear, fatigue or adhesion (14-micron and larger in the ISO 4406 codes).
It is, therefore, of the utmost importance to ensure that additional particles created during stress/load changes are removed as quickly as possible to limit the time in which they can damage gears, pumps, bearings, etc. — anywhere with an ultra-thin oil film clearances less than 3 microns. A particle wedged between moving surfaces creates hundreds of new particles, sending the wear in an increasingly vicious spiral.
Figure 7: Particle distribution curve for
abrasive wear, measured
with an online particle counter
An increase in the number of 14-micron and larger particles indicates an ”out of normal” operation, with possible severe abrasion, adhesion or fatigue propagation.
Testing of particle distribution in used oil (Figures 6-9) [9] shows that normal abrasive wear results in exponentially distributed particles with close to no particles of size 14 µm or above. Microscope inspection of the worn surface support wear scars below 5 µm.
When fatigue wear modes are investigated, the distribution curve changes and particles larger than 14 µm are detected in greater quantities. This is also supported by the visual surface damages that range from 50 µm to 100+ µm in diameter.
Figure 8: Microscope photo of severe wear.
Figure 9: Particle distribution curve
during abnormal wear generation (fatigue wear)
measured with an online particle sensor.
It is essential that the oil filters can keep up with the particles generated during operation. If the filters cannot, then the oil will get progressively more contaminated, resulting in reduced component and oil life.
The particle counts on WTG oils with a 3-micron CJC® offline filter installed [10] are typically ISO 15/13/10 according to ISO 4406 (see test results Figure 10).
Figure 10: Typical particle counts on WTGs with 3-micron CJC® offline filters (bottle sampling).
Particle counters typically use optical light extinction sensors [11], as do the online sensors in this study. The particle counts detect particles bigger or equal to 4 microns, bigger or equal to 6 microns and bigger or equal to 14 microns. The counts are converted into ISO codes/classes according to the ISO 4406 table (see Figure 11).
Figure 11: 4406 conversion table.
The online particle counters used in this study are Oil Contamination Monitors (OCMs)[12], which measure the particle counts for five minutes, averages them, and converts them into ISO codes; the cycle then repeats.
It is possible to see the ISO code trend as raw data or to smoothen data out over a 24-hour period.
Figure 12: Raw data of ISO codes for 10 WTGs between 2018 – 2022.
Looking at raw data over a long period, say four years, will impair the opportunity to see a trend, but averaging the ISO code raw data into 24 hours enables us to see the trend more easily.
Figure 13: Average 24-hour ISO codes for 10 WTGs between 2018 – 2022
Applying the Box Plot
A box plot model can be used to average the ISO codes over four years.
Here the meridian is indicated by the red line, and 50 percent of all data are in the blue box, while 99.3 percent of all data are within the whiskers indicated by black lines. Red points indicate outliers — measurements larger/smaller than 99.3 percent of the data.
Figure 14: Box plot model explained.
Applying the box plot model to ISO codes (4, 6 and 14-micron particles) for the 10 WTGs in this study:
Figure 15: Box plot of ISO codes for the 10 WTGs, separated into 4, 6 and 14-micron.
Figure 16: Average oil cleanliness ISO code for the 10 WTGs (4, 6 and 14-micron).
The wind turbine gear oils in this study are, in general, all super clean, with very few particles larger than 14 microns, meaning no indication of abnormal wear. The “normal” ISO codes for these 10 healthy WTGs sums up to be:
50 percent of the data are in the box from ISO code 10/7/1 up to ISO 14/11/6.
(ISO code 6 equals only 32-64 particles in 100 mL oil.)
99.3 percent of the data are within the whiskers from ISO code 4/1/0 to 20/14/15.
Alternatively, this can be written as: ISO 12/9/5 +/-2 (50 % of data) and ISO 12/9/5 +/- 8 (99.3 % of data).
Evaluating the 10 Wind Turbine Gear Oils in Terms of Oil Degradation
The same 10 wind turbine gearboxes were also fitted with online oil quality sensors, CJC® Oil Quality Monitors (OQMs), to evaluate the degradation state of the oils by means of resistivity.
All WTGs were using fully synthetic PAO-based oils, ISO VG 320, from well-known oil manufacturers (Castrol, Mobil, Fuchs and AMSOIL), which were anonymized in this study as oil types 1 to 5. The in-service life varies from three to more than 13 years, with two of the oils having an unknown age.
Figure 17: Oil types used in the 10 WTGs.
WTGs 4 to 8 are using the same oil type — type 4 — but with different in-service lives. WTGs 3 and 9 are also using the same oil type — type 3 — but at different ages.
The oil degradation/quality is monitored online every five minutes by the OQM sensors (resistivity). In the following illustration, the online data during the three years (2019-2022) are smoothened out over 24-hour periods:
Figure 18: Trend for oil degradation/quality (resistivity) for the 10 WTGs during 2019-2022.
Applying the box plot model to the resistivity data on the 10 WTGs reveals a drop for oil type 3 comparing the six-year-old to the 13+ years in service oils (WTGs number 3 and 9), but doesn’t show a severe drop in resistivity, so both oils are well maintained and don’t need to be replaced.
Figure 19: Comparing oil degradation/quality (resistivity) between WTGs number 3 and 9 with the same oil type, but different in-service life.
The five WTGs (numbers 4 to 8), which were using the same oil type (4), showed an even clearer picture of oil degradation/quality between the three-year-old and nine years in service oils.
Unfortunately, the age of the oil type 4 was unknown on WTG number 8, but since the resistivity has decreased so much, it is likely that the oil has been in service for many years, possibly more than 12 years.
Figure 20: Comparing oil degradation/quality (resistivity) between WTGs number 4 to 7 (8) with the same oil type but different in-service life
If not, then the oil has been poorly maintained and very contaminated most of its life prior to the offline filter installation.
Conclusion
The uptime of wind turbines depends on an efficient gearbox with clean oil. An offline oil filter (kidney loop) is operating continuously and should be able to remove the majority of newly generated particles. The CJC® offline oil filter has a very stable and consistent filtration efficiency and is able to keep up with particle generation, maintaining the WTG oil clean, dry and varnish-free. This has been proven for decades in more than 135,000 wind turbines worldwide, utilizing CJC® offline oil filters on gearboxes.
Learning Points From the Study
Regarding oil cleanliness in terms of particle counting:
• Healthy wind turbine gearboxes with well-filtered oil operate at around ISO 12/9/5 (+/-2)
• Trending on small (4-6 µm) particles will give insights to:
- Abnormal abrasive wear
• Abnormal operation of the filtration system and possible faults
• Trending on larger (14-40 µm) particles can give insight to:
- Severe abrasion/fatigue/adhesive wear
In terms of oil degradation/quality monitored by resistivity, the study shows:
• The state of oil degradation and aging can be trended
• Different oil types/brands have different levels of resistivity, so a sudden change could indicate wrong oil has been added
• Monitoring the oil resistivity trend can give insights to:
- Stability of the oil quality and state of degradation (possibly reduce oil sampling intervals)
- Remaining useful life of the oil in service
If you want to improve the power factor of your wind turbine, it is best done by keeping the oil clean using a combination of good air breathers, in-line oil filters and offline/kidney loop depth filters while monitoring and trending the oil cleanliness and state of degradation/quality.
Benefits When Improving Cleanliness and Monitoring the Oil for Particles and Oil Degradation/Quality:
- Increased operational reliability due to less component wear and better oil conditions
- Extended oil and machine component life due to less degradation
- Reduced oil consumption, resulting in savings and lower environmental impact (CO2 reduction)
- Less downtime, since problems can be foreseen and maintenance scheduled according to the data trend and wear situation
- Detection of abnormal operation, helping to find root causes more easily
- Detection of changes in oil quality, e.g., wrong oil added, which could cause foaming or short oil life
- Detection of abnormal wear. Replacing worn components proactively before a major breakdown occurs will yield large savings
Offline oil filters and online monitoring equipment do include initial costs but will result in large savings on the maintenance budget, plus an increase in the uptime and power factor for the wind turbine — offering a competitive advantage.
Online monitoring also adds additional safety and makes data trends readily available for interpretation.
References:
[1] R.S. Sayles, P.B. Macpherson, Influence of wear debris on rolling contact fatigue, Rolling contact fatigue testing of bearing steels. A symposium sponsored by ASTM committee A-1 on steel, stainless steel, and related alloys, ASTM STP 771 (1982) 255–274.
[2] Tormos, B. (2013). Engine Condition Monitoring Based on Oil Analysis. In: Wang, Q.J., Chung, YW. (eds) Encyclopedia of Tribology. Springer, Boston, MA. https://doi.org/10.1007/978-0-387-92897-5_1149
[3] Henneberg, M, Jørgensen, B. Eriksen, R. L. Oil condition monitoring of gears onboard ships using a regression approach for multivariate T2 control charts; Journal of Process Control 46 (2016) 1–10 http://dx.doi.org/10.1016/j.jprocont.2016.07.001
[4] Corugedo, Alexander & Pérez Barcala, Beatriz & Montero, Yosmari. The Electrochemical Impedance Spectroscopy as a Diagnostic Tool of the Transformer Oil. Revista Cubana de Ingeniería. 5. 10.1234/rci.v5i3.288.
[5] Henneberg, M. A method for Controlled Oxidation of Lube and Hydraulic
Oils and Investigation of the Effects on Oil Parameters ; Oildoc, 2015, January 27-29, (Proceedings), Rosenheim, Germany.
[6] Wei Cao, Han Zhang, Ning Wang, Hai Wen Wang, Zhong Xiao Peng, The gearbox wears state monitoring and evaluation based on on-line wear debris features, Wear, Volumes 426–427, Part B, 2019, Pages 1719-1728, ISSN 0043-1648, https://doi.org/10.1016/j.wear.2018.12.068.
[7] https://www.cjc.dk/products/t2render/, accessed 19-08-2022
[8] C.C.JENSEN A/S. Condition Monitoring Unit CMU, Advanced oil sensor system
Product Sheet. 2020. url: https : / / www . cjc . dk / fileadmin / root / File _
Admin_Filter/doc_Product_sheets/Monitoring_Equipment/ConditionMonitoring-Unit-CMU_PSMO4009UK.pdf.
[9] Henneberg, M. Eriksen, R. L., Tribological test and optical measurements of particles and their distribution as function of wear mode; Oildoc, 2017, January 24-26th, (Proceedings), Rosenheim Germany
[10] https://www.cjc.dk/products/fine-filters/hdu-27/, accessed 19-08-2022
[11] Krogsøe, K.; Henneberg, M.; Eriksen, R.L. Model of a Light Extinction Sensor for Assessing Wear Particle Distribution in a Lubricated Oil System. Sensors 2018, 18, 4091. https://doi.org/10.3390/s18124091
[12] https://www.cjc.dk/products/monitoring-equip/sensor-package/oil-contamination-monitor-ocm15-advanced-online-particle-counter/, accessed 19-08-2022
Illustrations:
illustration 1: Wind turbines in the ocean stock photo. iStock-1312167454.jpg
illustration 2 through 9, 12-13, plus 15-20: C.C.JENSEN,
Illustration 14: Box plot model explained. https://en.wikipedia.org/wiki/Box_plot
illustration 10-11: Oelcheck GmbH
For more information about CJC® offline oil filter systems, please visit C.C.JENSEN’s main website www.cjc.dk
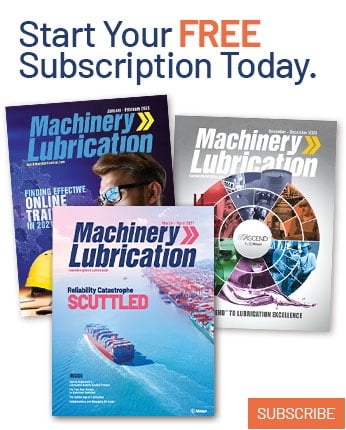