
Tests such as acid number (AN), Fourier transform infrared (FTIR), and the rotating pressure vessel oxidation test (RPVOT) should be commonplace in any well-designed oil analysis program, allowing oil analysis practitioners to scientifically determine when a condition-based oil change is warranted.
Conversely, little effort has been made to determine how similar analytical techniques can be applied to lubricating greases.
While it is a challenge to obtain a representative sample of in-service greases, simple scientific logic suggests that the rate of oxidation of a grease should be measurable to the oxidation of lubricating oils: after all, a grease has the same or similar additives as those found in conventional lubricating oils, bound up with a soap or other thickener.
The value of being able to scientifically monitor grease degradation is clear. While mechanical methods such as sonic and ultrasonic monitoring, shock pulse analysis and high-frequency vibration analysis have proven to be useful in assessing regreasing intervals, little work has been performed to assess the effects of base oil deterioration in greases.
However, with high-performance synthetic-based greases becoming increasingly commonplace and industry-leading companies demanding precision lubrication, being able to determine the optimum regrease interval may require paying closer attention to the oxidative and thermal performance of in-service greases.
Determining Grease Oxidation
To evaluate the effectiveness of oil analysis in monitoring the rate of thermal and oxidative degradation of lubricating greases, researchers at the Fundacion Tekniker in Bilbao, Spain devised a series of simple experiments to try to evaluate the effectiveness of some common and not-so-common oil analysis tests in determining grease degradation.
First, they took a conventional lithium grease formulated with a mineral base oil and typical additives found in most general-purpose greases. They then set about artificially inducing oxidation by stressing the grease over a five-hour period at 125°C.
Throughout this time, samples were taken at regular intervals and analyzed using a series of analytical methods. By comparing the test results, the research team drew some general conclusions concerning the effectiveness of the various test methods, which may contribute toward the use of these methods in the evaluation of in-service greases.
Testing Methods
To evaluate the degree to which the grease had oxidized, the team applied several different analytical techniques. First, solvent extraction was used to remove the base oil from the grease samples. After extraction, the following standard oil analysis tests were performed:
Kinematic viscosity
The viscosity of the base oil was determined at both 40°C and 100°C using ASTM D445.
Acid number
ASTM D974 was performed on the extracted base oil to determine the acid number (AN) of the oil/grease.
Infrared
After extracting the base oil using ether, a standard infrared spectrum was recorded (Figure 1). The team was particularly interested in the 1,700 to 1,780 cm-1 region, where infrared bands typically appear due to oil oxidation, as well as the 960 to 1,020 and 650 to 690 cm-1 regions, where absorption due to ZDDP, a common antioxidant and antiwear additive in many oils and greases, may be found.
Inductive coupled plasma spectroscopy (ASTM D 5185)
ICP is commonly used to determine wear metals contaminants and additive elements in used oil samples. The team focused on two elements, Zn and P, to try to measure the degree to which the ZDDP additive had degraded.
Figure 1. Infrared Spectra of the Extracted Oil Samples as a
Function of Oxidation Time
The goal of the team was to try to find the simplest, most cost-effective means of providing the information they were searching for. Therefore, they applied two less common techniques, specifically the RulerTM method and direct scanning calorimetry (DSC) in an attempt to evaluate the degree of oxidation.
RulerTM
The RulerTM instrument, discussed in a previous issue of POA, works on the principle of linear sweep cyclic voltammetry. By applying this test method, in which a variable voltage is applied to the sample while measuring the current flow, the presence and concentration of various antioxidant additives (including but not limited to ZDDP) can be determined based on their unique electrochemical oxidation potential and the magnitude of the induced current.
This procedure has recently been developed as a full ASTM test procedure under ASTM D6971. Because of its simplicity, this test was performed on both the extracted base oil and the grease (after suitable dilution).
Direct Scanning Calorimetry (ASTM D5483)
DSC has been used for several years to determine the degree to which greases have degraded. In this procedure, the grease is placed in a sealed vessel and subjected to heat in an oxygen-rich environment, similar to the more common RPVOT test.
By monitoring the temperature of the cell, the onset of oxidation can be determined when a sharp rise in heat generation is noted (commonly referred to as the induction point), due to the exothermic nature of the oxidation reaction.
The Results Are In
A summary of the results of this study are presented in Table 1. In general, most of the analytical techniques showed a significant change throughout the five-hour period when the grease was stressed, exhibiting excellent correlation between the methods. An exception to this are the viscosity readings at 40°C and 100°C, which are not particularly surprising because viscosity is known to be a lagging indicator of oil oxidation.
Table 1. Summary of Test Results
Of particular interest was the degree of correlation between data obtained using the RulerTM instrument on both the diluted grease and extracted oil samples, the infrared the AN readings measured in the oil, and the Zn and P concentrations from ICP (Figure 2).
Figure 2. Variation of Antioxidant Additives
(Measured by the RulerTM) and AN
Figure 2 confirms that as the oil degraded, a significant drop was observed in the ZDDP concentrations, as measured by the RulerTM instrument. At the same time, a reduction in the infrared peaks at 620 to 680 cm-1 and 970 to 1,080 cm-1 was also observed, along with a reduction in both Zn and P concentrations as determined by ICP, confirming the depletion of ZDDP.
The AN results were also interesting. The initial AN of the extracted oil was found to be approximately 2.4 mg KOH/g oil. As the oil starts to degrade, the AN dropped, particularly around the 80 to 90 minute mark when the other analytical techniques start to show a decrease (Figure 2).
However, after the other techniques indicated that the ZDDP was almost completely depleted (around the 100 to 150 minute mark), a slow but noticeable increase in AN was observed.
Again the conclusions are clear. The initial high AN of 2.4 in the new grease is largely due to the mildly acid nature of ZDDP, as measured by the AN test. As the ZDDP depletes due to thermal stress, the AN shows a corresponding drop consistent with this depletion. However, when the ZDDP, which in this case is serving as an antioxidant additive, is depleted it can no longer protect the base oil from oxidizing.
As the base oil begins to oxidize (around the 150 minute mark), the AN begins to rise as weak organic acid by-products of base oil oxidation are formed. A similar effect to this is often seen in ZDDP additized hydraulic fluids and other oils containing mildly acidic additives, and is again consistent with the other findings. Note that no change in viscosity was observed, despite the rise in AN.
Figure 3. Correlation between RulerTM
and DSC Results
The correlation of the DSC results with the other techniques was also apparent. Just like the RulerTM data, a clear drop in the DSC induction point was observed around the 80 to 90 minute mark (Figure 3). It remains that DSC is an excellent technique alongside the other methods used in this study to detect the onset of grease degradation.
Conclusions
Based on the results, the team was able to draw some general conclusions regarding the efficacy of the various techniques in monitoring grease degradation:
-
The RulerTM instrument was able to offer a simple field method, which did not require the oil to be extracted from the grease (a time-consuming and complicated process).
-
While ICP proved effective at monitoring ZDDP depletion in this case, it may not be possible to use this technique where no organometallic additives are present.
-
Infrared is an effective technique in determining antioxidant additives, provided the absorption bands lie in a relatively clear area of the spectrum (as in this case), and that a new oil base line is available to quantify the degree of infrared absorption of the new grease and the appropriate resonant frequencies.
-
AN and particularly viscosity measurements are less effective in determining oxidation potential than other techniques (such as infrared and RulerTM) that directly measure additive concentrations.
-
The correlation between RulerTM and DSC methods appears to be excellent though further studies may be necessary to recognize if this is applicable in all cases.
-
Complete depletion of ZDDP appeared to correlate to an approximate 50 percent reduction in Zn and P concentrations in the extracted oil samples, due to the presence of by-products and ZDDP depletion that remain in the oil and still contain elemental Zn and P.
Author’s Note:
This article is based on a technical paper presented at the 17th ELGI AGM in Edinburgh, Scotland in May 2005. Download the full article - PDF (1MB)
Related Reading
William R. Herguth and Howard Adams. “Sunlight Degradation of Lubricants.” Practicing Oil Analysis magazine, September-October 2005.
“The Ruler.” Practicing Oil Analysis magazine, July 1998.
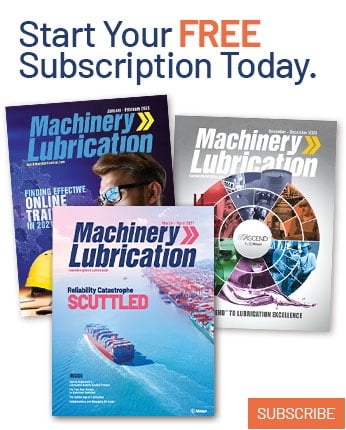