It is widely believed that extreme conditions are uncommon; however nearly every manufacturing plant has at least one application in which the operating conditions could be characterized as extreme from a lubrication perspective. Common extremes could include high and low shaft speeds, high and low temperatures, high pressures, concentrated atmospheric and process contaminants, and inaccessibility.
Mineral oil-based fluid lubricants (oil and grease materials) function properly where the designed surface areas and shaft speeds allow for the effective formation of an oil film, as long as the machine operating temperature envelope falls between -20°C and 100°C (-4°F to 212°F).
The only absolute limits that apply for fluid lubricants, regardless of the base oil type, are conditions that cause a change in the state of the fluid that prohibits fluid film formation. Fortunately, that is not the end of the story.
Various materials that protect interacting surfaces after the fluid film is lost have been either discovered or created. These materials may be applied to a surface in the form of an additive to a fluid lubricant, or in a pure form, and may also be added or alloyed into the surface when the component is being manufactured. The more common types of materials include the following:
- Molybdenum disulfide (MoS2) – also known as moly
- Polytetrafluoroethylene (PTFE) – also known as Teflon®
- Graphite
- Boron nitride
- Talc
- Calcium fluoride
- Cerium fluoride
- Tungsten disulfide
These materials are characterized as dry film or solid film lubricants. Moly, graphite and Teflon are the most commonly recognized by practitioners of machinery lubrication. Molybdenum and graphite are agents that are extracted from mined ore. Teflon was created by DuPont Chemical Company and is manufactured by various companies for many purposes.
Dry Film Lubrication
Dry film lubricants are solid materials that provide low frictional resistance between surfaces when applied directly to interacting surfaces. Each material has different properties. Crystalline lattice (lamella) structure materials, such as molybdenum disulfide, tungsten disulfide and graphite, are widely used as agents in lubricants and as stand-alone lubricants.
These materials are used independently or in combination with other agents and metals (lead, copper) to achieve the desired results. Lamella lubricating powders have low shear forces between their crystalline lattice layers that minimize resistance between sliding surfaces.
Figure 1. Crystal Structure of MoS2
(Reference: Dynamic Coating, Inc.)
As seen in Figure 1, these materials have structured layers that form and interact against other structure layers. Most dry lubrication film materials work well in dry environments and are excellent supplemental or boundary layer materials in fluid systems.1
The long chain fluorocarbon molecules, such as polytetrafluoroethylene, tend to have wetting angles that promote release and prevent sticking, as well as a variety of other attractive characteristics for high-temperature operation. This article addresses the most frequently used dry film lubricating agents.
General Dry Lubricant Properties
Each dry lubricating material has different properties.
Molybdenum disulfide, graphite and tungsten disulfide are oilioscopic. Their structure is unable to tolerate detergents. These layer lattice lamella structures are analogous to stacks of nonadherent plates which, with slight tangential loading, slip out of place.
It is comparable to walking across a room full of flat slippery plates. The lamella materials have good load-bearing capacity in sliding and rolling mode. Graphite has high-temperature capability and functions well in radiation atmospheres. MoS2 and WS2 function well in hard vacuum and tolerate higher loads better than graphite.2
Molybdenum Disulfide (MoS2)
Molybdenum was not discovered until the latter part of the 18th century, and does not occur in the metallic form in nature. Despite this, its predominant mineral - molybdenite - was used in ancient times (a 14th-century Japanese sword has been found to contain molybdenum) but would have been indistinguishable from other similar materials such as lead, galena and graphite. Collectively, these substances were known by the Greek word “molybdos”, which means lead-like.
Molybdenum was first positively identified in 1778 by a Swedish scientist, Carl Wilhelm Scheele. Shortly thereafter, in 1782, Peter Jacob Hjelm reduced molybdenite oxide with carbon to obtain a dark metallic powder which he named “molybdenum”.
Molybdenum remained a laboratory curiosity throughout most of the 19th century until the technology for the extraction of commercial quantities became practical. In 1891, the French company Schneider and Co. first used molybdenum as an alloying element in the production of armor plates. It was quickly noted that molybdenum was an effective replacement for tungsten in numerous steel alloying applications because its density is only slightly more than half that of tungsten.
MoS2 occurs naturally in the form of thin solid veins within granite. It is mined and highly refined to achieve purity suitable for lubricants. MoS2 has a hexagonal crystalline structure as shown in Figure 1. The intrinsic property of easy shear occurs at the interface between the sulfur molecules.
The interaction between layers provides an effect similar to what a person may experience if trying to maneuver across a floor completely covered with brand new playing cards. Each playing card slides easily with respect to each individual layer. As the total surface resistance is reduced or redistributed among many other interacting surfaces, the net effect is reduced total surface friction and resistance.
Because there is no vapor present between lattice plates, MoS2 is effective in high-vacuum conditions, where graphite will not work. The particle size and film thickness are important parameters that should be matched to the surface roughness of the lubricated component.
Particle size selection is much larger for rough cut surfaces, such as hobbed open gears, than for highly finished surfaces, such as those found on bearings. Improperly matched particle sizes may result in excessive wear by abrasion caused by impurities in the MoS2.
The temperature limitation of MoS2 at 400°C (752°F) is imposed by oxidation. MoS2 oxidizes slowly in atmospheres up to 600°F. In a dry, oxygen-free atmosphere it can function as a lubricant up to 1300°F. The oxidation products of MoS2 are molybdenum trioxide (MoO3) and sulfur dioxide. MoS3 is hydroscopic and causes many of the friction problems in standard atmosphere. MoO3 is a preferred form of the metal used as an additive for various other metals, which is its primary use.
The issue of where molybdenum disulfide should be used, versus graphite or tungsten disulfide, is generally best addressed by a lubrication engineer. For most commercial applications, these are relatively simple judgments. In aerospace applications where unique environments and exotic materials are employed, these questions often take substantial research to provide the best answers.3
The low friction coefficients of MoS2 often exceed that of graphite.
Graphite
Graphite as a lubricant dates to antiquity. It was first referenced in the mid-1500s as being used as pencils. Graphite is a soft, crystalline form of carbon. It is gray to black, opaque, has a metallic luster, and is flexible but not elastic. Graphite occurs naturally in metamorphic rocks such as marble, schist and gneiss. It exhibits the properties of a metal and a nonmetal, which makes it suitable for many industrial applications.
The metallic properties include thermal and electrical conductivity. The nonmetallic properties include inertness, high thermal resistance and lubricity. Some of the major end uses of graphite are in high-temperature lubricants, brushes for electrical motors, friction materials, and battery and fuel cells.4
Graphite is a layer lattice lamella crystal structure where the bonds between the carbon atoms in the crystal structure of the layer are stronger than the carbon bonds between layers. Graphite is comprised of carbon and water vapor. Each carbon atom is bonded to three other surrounding carbon atoms.
The flat rings of carbon atoms are bonded into hexagonal structures, as shown in Figure 2. The hexagonal carbon structure is referred to as a benzene ring. These plates exist in layers, which are not covalently connected to the surrounding layers.
Figure 2. Graphite Lamella Lattice Structure
(Reference: Dynamic Coating, Inc.)
Graphite has excellent lubricating properties, as long as moisture vapor is available, and will function as a lubricant up to approximately 1450°F, and as a release and antiseize up to about 2400°F. The oxidation product is CO2. Due to the requirement for entrained moisture vapor, graphite does not function well as a lubricant in a hard vacuum and is therefore seldom used in deep-space applications.5
Graphite blends and pure graphite dry film lubricant systems are commonly used in applications such as hot and cold forming, wire drawing and billet coatings; on high-speed cutting tools; as a mold release for die cast, plastic and rubber mold applications; cylinder head and exhaust bolts; ammunition and armament applications; automotive engine and many common industrial applications.
Figure 3. Graphite Ore
Figure 4. Teflon Molecular Structure
Long Chain Fluorocarbon - Teflon®
The linear long chain molecule “polytetrafluoroethylene”, was accidentally discovered by Dr. Roy Plunkett on April 6, 1938, at DuPont’s Jackson Laboratory in New Jersey. Plunkett was working with gases related to Freon® refrigerants (also known as chlorofluorocarbons), another DuPont product. Upon checking a frozen, compressed sample of tetrafluoroethylene, he discovered that the sample had polymerized spontaneously into a white, waxy solid to form polytetrafluoroethylene (PTFE).6
The chemical formula is [C2F4] N. PTFE is a saturated aliphatic fluorocarbon.
Figure 5
Figure 6
PTFE does not have mechanical occlusion characteristics similar to graphite or molybdenum. In fact, Teflon itself resists wetting, and the surfaces coated with these materials likewise resist wetting. For surfaces to bond with PTFE and the variety of other long chain fluorocarbons, it is necessary for them to be properly prepared through surface roughing or pickling.
PTFE is inert to virtually all chemicals and is considered the most slippery material in existence. The coefficient of static and dynamic friction is nearly equal to the level of wet ice on wet ice. As DuPont registered trademark Teflon®, it has become a household name associated with its use as a coating on cookware and as a soil and stain repellant for fabrics and textile products. It does not absorb water, and is unaffected by acids, bases and solvents normal to industry at temperatures less than 500°F.
Figure 7
Various fillers can be added to PTFE to enhance certain characteristics, such as glass fiber (high wear resistance, good electrical, low friction), graphite (low friction, excellent chemical resistance, high creep resistance), carbon fiber (high wear resistance, high load resistance, high bend resistance), glass fiber and molybdenum disulfide (high wear resistance, low friction, high creep resistance), and bronze (high wear resistance, heat dissipation).
In some industries, such as the bearing pad industry, filled PTFE has become the standard, where various percentages of glass fiber are added to the base PTFE resin to create an extremely tough, weatherproof, interface material that can be cut or stamped into configurations to match the dimensions of the opposing surfaces.7 PTFE is licensed to many manufacturing firms for a variety of material types.
Solid film lubricants offer protection beyond the normal properties of most mineral and synthetic oil-based fluid lubricants. Conditions that warrant the use of these agents in a pure form, or as an additive, include extremes of temperature, pressure, chemical and environmental contamination.
Some agents have a strong affinity for metallic surfaces, and will adhere to those surfaces through loose covalent forces. These may be applied directly as a topical coating or indirectly in the form of an additive to a fluid lubricant. Some agents have no natural attractiveness to metallic surfaces, and therefore must be bonded to the surface through specialized treatment.
The solid lubricating materials tend to have upper temperature ranges well above the surface-protecting capabilities of mineral and most synthetic base stocks. Fluorinated hydrocarbons are stable in liquid or solid form to roughly 600°F, but will begin to degrade and may produce noxious fumes at that temperature.
Graphite and molybdenum can operate in a similar temperature range, and molybdenum disulfide can also function in a vacuum without losing its slippery property.
References
-
Kleimann, Colonel. Dynamic Coatings, Inc.
-
Kleimann, Colonel. Dynamic Coatings, Inc.
-
Climax Molybdenum Company.
-
Kleimann, Colonel. Dynamic Coatings, Inc.
-
U.S. Geological Survey.
-
Dupont Corporation.
-
Fluoropolymer Products.
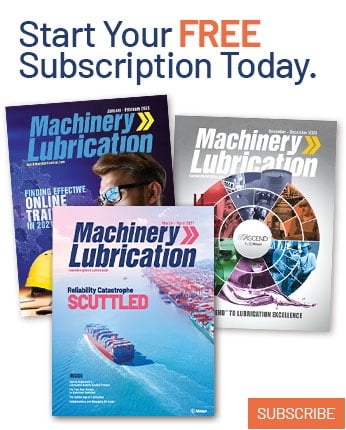